THE DIFFUSION OF SULFUR DIOXIDE IN AIR THROUGH STACKED LAYERS OF PAPER
Michael A. Dimitroff, & James W. Lacksonen
ABSTRACT—Sulfur dioxide (0.5% to 1.0%) in air at 73�F and 57% relative humidity was used to measure the effective diffusivity of sulfur dioxide through paper. A 3.8cm thick block of stacked layers of 100% cotton bond Crane's Kid Finish in Pearl White color was mounted in a diffusion cell. Diffusion studies were made in directions both normal (perpendicular) and parallel to stacked sheets of paper. An average effective diffusivity of 0.0072 cm2/s and a tortuosity of 4.7 were calculated for the diffusion of sulfur dioxide normal to the paper. An average effective diffusivity of 0.036 cm2/s and a tortuosity of 1.0 were calculated for diffusion parallel to the paper.
The diffusion, absorption and reaction of various gases in paper can be important to both the degradation and preservation of paper objects. Some objects, such as books, can be thick compared to a single sheet of paper. The rate of penetration (by diffusion) of gases into thick paper objects has apparently not been studied as completely as absorption studies with single sheets, although early work by Jarrell, Hankins and Veitch1 did show the significance of different kinds of papers, humidity and metal ion additives in a 15 sheet stacked sample simulating a book.
Other researchers2, 3 used radioactive tracer techniques to measure low concentration absorption of sulfur dioxide in single sheets of 100% rag paper and 6% groundwood, 40% chemical wood printing paper. Sulfur dioxide concentrations from 5000 to 0.75 ppm were used. The conclusion was that humidity affected the short term rate of absorption but not the long term rate. A reversible equilibrium followed by irreversible effects was postulated to explain the results. A number of investigations3, 4, 5 have been made which studied the effects of catalytic and inhibitor additives. The rate of sulfur dioxide fixation was increased by copper and manganese; iron showed a low catalytic effect, perhaps because it was not present as a soluble salt. In a study4 of the combined effects of metallic catalysts and inhibitors (EDTA, sodium phosphates, 8-hydroxyquinoline sodium citrate and ammonium oxalate), the catalytic effects were greater than the inhibitor influence.
Studies made on the transport of water vapor through paper suggest7 that water vapor permeabilities are exponentially related to the relative humidity. The adsorption-desorption of water vapor was modelled as pseudo-first order kinetics.8 The rates were dependent on the history of the sample.
It appears that the diffusion phenomena have not been specifically studied, with perhaps the exception of water vapor. The degradation of paper by acids is well-documented. With the present problems of air pollution, acid rain, etc., it seemed that a study of the acid gas sulfur dioxide would be of general interest, particularly to those concerned with large collections of thick paper objects often housed in metropolitan areas where air pollution can be most severe. Therefore, the sulfur dioxide-air system was selected for study.
The use of 0.5% to 1% sulfur dioxide in air is considered a “dilute” gas, although it is many times more concentrated than the few ppm typical of sulfur dioxide concentrations in city air. The higher concentration levels were dictated by limited sensitivity of the available gas chromatographic technique. Generally, low concentration processes are linearly dependent on concentration. This permits convenient extrapolation to lower values. In a sense, the “higher than normal” sulfur dioxide concentration used is akin to accelerated aging tests done on paper at elevated temperatures. Also, the objective of this phase of the work was to evaluate the effective diffusivity of sulfur dioxide at steady-state conditions. From previous studies in the area of gas diffusion,11 there is evidence to suggest that a 1% gas phase concentration can be considered as “dilute” and any further reduction in concentration should not provide any new or unusual diffusion phenomena.
2 EXPERIMENTAL
2.1 Analytic Equipment
A Sargent-Welch gas chromatograph model S-38585 was used for the gas analysis of sulfur dioxide in air. Accurate readings to 0.01% sulfur dioxide in air could be made with a 1 ml. sample. The thermoconductivity detector with helium carrier gas was used with a Supelpak-S column (a porous polymer packing of Porapak-Q type that is acid washed). Pure sulfur dioxide was used to prepare calibration samples with calibrated gas burettes.
2.2 The Diffusion Cell
A diffusion cell similar to that used to measure diffusivities in porous catalysts9 was constructed from 6 inch, schedule 80 PVC plastic pipe and glass. Two chambers were separated by a block of paper through which the gas of interest would diffuse. Each chamber was 310 cm3 in volume and was fitted with a plastic impeller which was magnetically driven to insure complete mixing. The paper block between the chambers was 10.0 cm by 10.0 cm (100 cm2 area) and was mounted in polyethylene 3.8 cm thick. Each chamber had � inch entrance and exit lines for admitting and removing gases.
2.3 The Paper Block
The paper used in this study was 100% cotton fiber bond Crane's Kid Finish in Pearl White color. The cold extraction pH of the paper (TAPPI T428) was 5.1.
Different orientations of the paper sheets were used in this study. For diffusion normal (perpendicular) to the stacked sheets, 226 sheets were stacked with the machine direction parallel, clamped between two pieces of Plexiglas, and silicone rubber cemented on the edges. There was no seepage of cement into the block. The block was mounted into the test section of polyethylene with silicone rubber cement. To avoid bulging, 11 cm. by 11 cm. sheets of similar paper were cemented to the top and bottom faces of the test section and weighted during drying. The block of paper gave an average of 59.22 sheets per cm of thickness. For diffusion parallel to the sheets of paper, similar construction methods were used. Five hundred ninety-two sheets 10.05 cm by 3.80 were cut with the width dimension being the machine direction. Since bulging was not a problem when this block of paper was mounted in the test section, the two extra sheets on the top and bottom were not used. The paper block test sections could easily be removed with no damage by strongly pushing it out by hand. After the diffusion studies were completed, the porosity of the paper blocks was determined.
2.4 Experimental Setup and Operation
The experimental setup used to make the diffusivity measurements is shown in Figure 1. The air to the system was filtered and humidified by passing it through an aqueous solution of 83.6 wt % glycerine. Humidification measurements showed the air was 57% relative humidity at 73�F. Sulfur dioxide of 99.995% purity could be mixed with part of the inlet air stream to any desired composition. The top chamber of the diffusion cell had only humidified air entering, while the bottom chamber had humidified air with sulfur dioxide. The paper was “conditioned” in the cell for three days with humidified air and for an additional 36 hours with sulfur dioxide at the desired concentration to establish local equilibrium with the paper. (Previous separate absorption studies of sulfur dioxide with the paper had been done to insure that 36 hours gave no detectable change in absorbed sulfur dioxide.) After the paper was conditioned, it was considered to be at steady-state and 1.00 ml gas samples were taken from the exit stream of each chamber. Samples were taken every 15 minutes for 2.5 hours, followed by an 8 hour wait, then another set of samples was taken. Air flow rates were periodically measured with the soap-bubble flow meter. The chambers were operated at constant and equal pressure so that sulfur dioxide passed through the paper into the upper chamber by diffusion only. The steady-state diffusion rate of sulfur dioxide could be calculated since the flow rates and concentrations in the chambers were measured. The exiting gases were bubbled through two sodium hydroxide solution scrubbers in series to remove sulfur dioxide prior to venting the gases.
Fig. 1.
Apparatus Used for the Steady-State Diffusion of Sulfur Dioxide Through Stacked Layers of Paper, Schematic Diagram
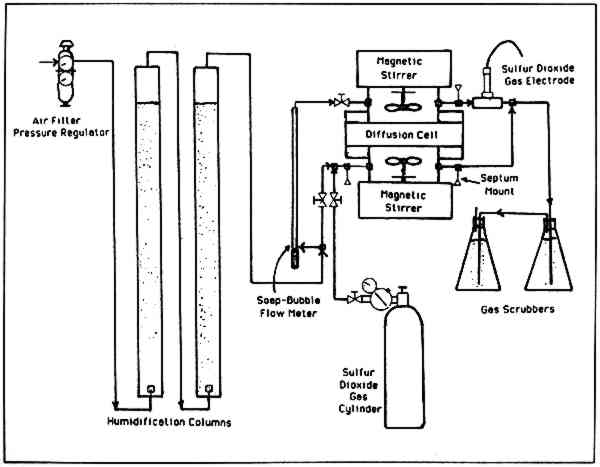 |
3 RESULTS
The determination of the effective diffusivity was made by coupling Fick's First Law with the sulfur dioxide material balance over the top chamber where sulfur dioxide was entering by diffusion through the paper block. Under steady-state conditions, Fick's First Law can be assumed as:
Fig. .
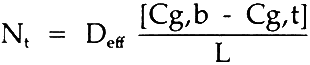 |
where:
Nt= molar flux of sulfur dioxide through the paper; Cg,b= molar concentration of sulfur dioxide, bottom; Cg,t= molar concentration of sulfur dioxide, top; L= diffusion length (block thickness); Deff= effective diffusivity.
The sulfur dioxide material balance over the top chamber is:
Fig. .
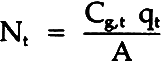 |
where:
qt= volumetric flow rate of gas out of top chamber A= area of diffusion
Combining these equations gives:
Fig. .
 |
Where Y represents the mole fraction or mole percent of sulfur dioxide. For this study, A = 100 cm2, L = 3.8 cm and qt and Y values were averaged for the different runs. For diffusion normal to the paper, the average diffusivity was found to be 0.0072 cm2s and for diffusion parallel to the paper, it was 0.036 cm2/s. Table 1 summarizes the experimental data used to calculate the diffusivity values.
TABLE 1 Effective Diffusivity Components of Sulfur Dioxide in Air Through Stacked Layers of Paper, Experimental Results For Diffusion Parallel to the Stacked Sheets of Paper Due to a Concentration Gradient in the Same Direction
The tortuosity of the paper block is defined by the parallel pore model equation:10
Fig. .
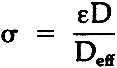 |
where:
ω= tortuosity ε= porosity D= binary gas phase diffusivity Deff= effective diffusivity in porous medium.
Tortuosity represents the resistance to diffusion through a porous solid due to the influence of the pore structure. It assumes that a “tortuous path” delays the diffusion of a component compared to a “straight line” path. The concept of tortuosity is a mathematical and conceptual description that can help visualize the importance of the structure to diffusional processes. In order to find the tortuosity, the porosity had to be found. This was done by placing the weighed block of paper in a 2-liter beaker. Silicone oil was added and the beaker was put in a vacuum oven for three hours. The silicone oil replaced the air in the void spaces. The paper block was removed and weighed. From the density of the oil and the weight drawn into the block, the porosity of the paper block was found to be 0.26. Literature values for the binary gas diffusion coefficient for sulfur dioxide in air were corrected to the conditions of this experiment and used to find the tortuosity. Diffusion normal to the paper block gave a tortuosity of 4.7 whereas diffusion parallel to the paper gave a tortuosity of 1.0.
4 CONCLUSIONS
Our research into the sorption/diffusion of gases into paper has concentrated on the steady-state evaluation of the effective diffusivity and tortuosity of sulfur dioxide in air. It can be argued that although we have thus far studied only one paper, the physical properties of the paper as prepared in a multilayered block should be reasonably similar to many other papers. With respect to the diffusion processes, it is significant to note that the diffusivity was about five times greater in the parallel direction compared to the normal direction. Of greater importance is the fact that the tortuosity in the parallel direction is 1.0 for this system. It's as if there were no resistance from the paper. This is equivalent to diffusion between parallel plates. Note also that the tortuosity calculation compares the effective diffusivity with the binary gas phase diffusivity. If the distance between the sheets were very small (less than one tenth the mean free path of the gas), than Knudsen diffusion would be expected. This apparently is not the case and the diffusion in the parallel direction is like diffusion through almost straight macropores. Since the paper blocks were prepared to simulate books, it is likely that diffusion into books via the parallel direction should be analogous to this study. Diffusion in the normal direction is more likely to be different and more dependent on the kind of paper, although the magnitude of this difference has yet to be determined.
It is suggested that suitable models for the diffusion of gases into books or documents which have a reasonable number of pages can be handled by assuming the pages are like parallel flat plates. Coupling the sorption phenomena to this model for the unsteady-state sorption diffusion process in one direction would require solving the differential equation (probably via computer)
Fig. .
 |
where:
εp= porosity of stacked paper Cg= concentration of gas X= distance into paper R= sorption rate equation, dependent on gas phase concentration Cg and absorbed phase concentration Cp
Preliminary sorption research has been done by the authors with the system described here. We have found that the sorption/desorption process is considerably more complex than the diffusion phenomena and future research should dedicate more effort to understanding this part of the penetration of gases into multilayers of paper.
REFERENCES
T.D.Jarrell, J.M.Hankins and F.P.Veitch, Deterioration of Paper as Indicated by Gas Chamber Tests, U.S. Dept. of Agriculture Technical Bulletin No. 605, Washington D. C.: U.S. Govt. Printing Office, Jan. (1938).
F.L.Hudson, R.L.Grant and J.A.Hockey, “The Pickup of Sulfur Dioxide by Paper,” J. of Applied Chem., (1964), 14: 444–47.
C.J.Edwards, F.L.Hudson and J.A.Hockey, “Sorption of Sulfur Dioxide by Paper,” J. of Applied Chem., May (1968), 18: 146–8.
F.L.Hudson and W.D.Milner, “The Permanence of Paper-The Use of Radioactive Sulfur to Study the Pickup of Sulfur Dioxide by Paper,” Paper Technol., 2 (1961): 155–61.
J.B.Atherton, F.L.Hudson, J.A.Hockey, “The Effect of Temperature, Light and Some Transition Metal Ions on the Sorption of Sulfur Dioxide by Paper,” J. of Applied Chem. and Biotechnol., 23 (1973) 407–14.
A.T.Ahlen, “Diffusion of Solid Water Vapor Through Papers and Cellulose Films,” TAPPI47, F(1964): 95–98.
R.R.Rounsley, “Vapor Transport Through Paper,” TAPPI, 47 F(1964): 95–8.
S.Brooks, “Kinetic Sorption and Desorption of Water Vapor,” TAPPI, 50 Aug. (1967) 103A–8A.
J.M.Smith, Chemical Engineering Kinetics, 3rd Ed., McGraw-Hill (1981): 462–3.
Ibid., 465.
E.L.Cussler, Diffusion: Mass Transfer in Fluid Systems, Cambridge U. Press (1984).
Section Index |