THE RECOVERY AND DRYING OF TEXTILES FROM A DEEP OCEAN HISTORIC SHIPWRECK
KATHRYN A. JAKES, & JOHN C. MITCHELL
ABSTRACT—A trunk full of waterlogged and degraded textiles recovered from the site of S.S. Central America, which sank off the coast of the Carolinas in 1857, provided the opportunity to conduct research in the identification and characterization of such materials as well as in the appropriate methods for their conservation. The plan included removal of each item from the trunk, immersion in demineralized water, assessment of each item, arrangement on fiberglass screens, and rapid freezing. Experiments conducted to study the effects of air drying, ethanol dehydration, critical-point drying, vacuum freeze drying, and slow drying in the frozen state revealed vacuum freeze drying to be the method most disruptive to fabric and fiber structure. Slow drying while in the frozen state resulted in fibers and fabrics with visible structure and few contaminating deposits on the surface.
1 INTRODUCTION
So rarely are historic textiles retrieved from long-term storage in the marine environment that little is known about the degradation processes they have undergone, the consequent chemical and physical structure of the degraded materials, and the appropriate methods for their conservation. The fiber content of some marine textiles has been identified, for example, the wool and linen from the Mary Rose has been described by Ryder and Gabra-Sanders (1985). Their methods were limited to microscopy and scanning electron microscopy; although the fibers were identified, their chemical macromolecular physical structure was not characterized. The difficulty of identifying certain historic and archaeological textile materials of any provenance is noted by many authors (King 1978; Schaffer 1981; Goodway 1987). Techniques that characterize archaeological textile materials have been undertaken in few cases, none with examples from the marine environment (Jakes and Sibley 1989; Jakes and Angel 1989).
So little is known about the degradation of textiles in marine environments that conservation of the few examples that have been found has taken an experimental approach—sometimes successful, sometimes unsuccessful. Somewhat more research has been conducted on waterlogged wood. Such treatments may suggest possible routes for treatment of textiles (e.g., Rosenquist 1975; McCawley 1977; Grattan 1989), but the difference in bulk between the two will dictate some differences in handling methods. For example, wood artifacts contain internally adsorbed water, which is not removed by normal sublimation of ice in freeze drying (Rosenquist 1975). As a result, differential drying and shrinkage of the piece occur. Textiles, on the other hand, have less bulk and a large surface area; ice within the textiles is readily accessible to sublimation through freeze drying. In addition, treatments useful for drying waterlogged wood may not be directly translatable to textiles due to the effects of processing, dyeing, and other treatments, which increase the complexity of textiles and complicate the prescription of the appropriate handling methods.
Pearson (1987) describes work done with archaeological marine textiles in the categories of drying from water, from other liquids, with consolidants, with bulking agents, with lubricants, and by sublimation. Mayhew's review (1990) compiles some of the literature on waterlogged textile materials. Drying from water is the most desirable and least intrusive method if no fiber shrinkage or embrittlement occur. Drying from other liquids can reduce fiber collapse on drying but can also remove components of the textile such as dyes, pigments, or fats and oils. Bengtsson (1975) dried sails and ropes from the Wasa by extracting the water with alcohol followed by xylene. Xylene was used to allow fabric drying without shrinkage, but the resulting textiles were brittle and fragile.
Drying with consolidants such as polyvinyl acetate and bulking agents such as polyethylene glycol has been reported. Grosso (1975) cleaned historic textiles with soap, then mounted the fragments on glass using a polyvinyl alcohol solution. Morris and Seifert (1978) used a polyethylene glycol consolidant prior to vacuum freeze drying of a shot bag from the Defence. Leather objects have been successfully treated by freeze drying them with a glycerol lubricant (David 1981; Ganiaris et al. 1982), and Pearson (1987) suggests the adoption of this method for textiles.
Norton (1990) reports on conservation treatments for archaeological and historic objects made from plant materials. She states that the use of water alone is sufficient to cause damage in materials. Polyethylene glycol lubrication can discolor with time. Consolidants can darken and gloss with time, and they can stiffen the fabric. Some consolidants can contribute to artifact degradation.
Nonalteration of the basic fabric is the goal of the conservator; some care should be given in choice of treatments since so little is known about the long-term effects of those treatments. Pearson (1987) notes one personal communication that states the effectiveness of slow drying from the frozen state in the drying of archaeological textiles.
Peacock (1990) addresses the need for basic research on the use of vacuum freeze drying with archaeological textiles. While vacuum freeze drying has been employed in isolated cases, she cites many problems with these studies, including the fact that despite the use of a variety of pretreatments, no research has been reported concerning the compatibility of these treatments with the fibers and fabrics treated.
Conservators agree that treatments traditionally employed and generally accepted may not be as successful as they first appeared. A recent symposium of textile conservators addressed these issues (Harpers Ferry Regional Textile Group 1986). Even the longstanding procedure of needle stitching support fabrics to fragile textiles was questioned at this symposium.
Since the opportunity existed to work with a large number of waterlogged textiles of known provenance, a research plan was developed that contributes to the knowledge of waterlogged historic textiles and their conservation. The quantity of waterlogged textiles available allowed some to be used for experimentation. In this paper, a salvage operation is described in which the textiles were frozen to halt degradation. The subsequent evaluation of appropriate drying methods for these waterlogged textiles is reported.
2 HISTORY
In 1857, the steamship Central America sank off the coast of the Carolinas due to damage suffered in a hurricane. Four hundred twenty five passengers died in the catastrophe; 153 survived. The ship had been traveling from the Isthmus of Panama to New York carrying passengers and a shipment of gold for the New York banks. Newspapers carried stories of the survivors for many weeks; the history of the accident has been detailed in Story of an American Tragedy(Conrad 1988; see also Conrad 1991). Using sophisticated side-scan sonar technology and probability mapping of the location of the Central America, the Columbus-America Discovery Group located the ship in 1987 and has continued to study the wreckage and the surrounding area each year (Herdendorf 1991). As the site surrounding the wreckage was explored, items were found strewn across the ocean floor. A trunk was examined underwater in 1988. As a jet of water was blown on the latch to see what was written there, the trunk fell open. Each half was covered with a fabric flap or baffle as suitcases are today. These baffles held the clothing within the trunk even when the trunk was opened underwater. The trunk lay in this configuration until September 1990, when it was retrieved. The trunk halves were placed in Plexiglas tanks, lifted with the deep-sea diving submersible vessel called Nemo, and taken to Columbus, Ohio, in October. Examination of a few items of clothing from the trunk showed them to be contiguous and recognizable (Lore 1990; Ringle 1990).
3 RECOVERY OPERATION
Although the Plexiglas tanks containing the trunk halves were lined with trapaulins to reduce water loss, water had to be added to the tanks during the transport of the trunk to Columbus, where the trunk halves were stored temporarily in a warehouse. A few items were taken out of the trunk and unrolled. These garments appeared contiguous and withstood the operation of unrolling without obvious damage. They quickly were reimmersed until a plan was devised for their care. When it was discovered that the contents were garments in recognizable condition, the Department of Textiles and Clothing at Ohio State University was contacted and an emergency salvage plan was initiated. The trunk obviously contained a large quantity of items, but they were submerged in black water. The change in ionic state of the water in the tanks as tap water was added in order to keep the contents submerged was a concern, as was the much warmer temperatures of the storage environment compared to that in the deep ocean. Not only could some structural change occur as the water conditions changed, but microbial activity could be much increased. Given the need to assess the items in the trunk and the possible danger of their deterioration in the storage environment, a plan for freezing the garments was devised and carried out.
On November 5 and 6, 1990, the items were assessed and frozen. Each garment was lifted from the black water within the trunk and unrolled while submerged in demineralized water in a large tank. The items were kept wet at all times because the plasticized state helped minimize damage during unrolling and arrangement. The tanks in which the garments were unrolled were refilled with clean water for the immersion of each item; after removal of the item, samples of water were taken for later pH measurement. Each item was numbered and photographed, and an inventory was made. For future fiber identification, small samples were taken from inconspicuous locations, such as inside seams. Some garments were not sampled, either because the information would be redundant (e.g., only one sample was taken from nine handkerchiefs of the same construction) or because the item of clothing appeared to be so special that sampling would be damaging (e.g., a silklike scarf that was hand hemmed on all four sides). Each garment was arranged underwater on a fiberglass screen, raised from the water, allowed to drain briefly, and placed on another screen stretched over a wooden 6 � 3 ft frame. Each garment was arranged to provide a thin flat surface that would freeze quickly and to provide a pleasing garment configuration in the event that the item would not be flexible after it was dried. The framed screens were placed on racks in a freezer at −19�F. Rapid freezing of the water within the textile was desirable, so that smaller ice crystals would result. Slow cooling allows molecules to align themselves, thus creating large crystals (Mellor 1978). Smaller crystals would be desirable because they would be potentially less disruptive of the fiber structure. Thin garments were frozen as quickly as they were brought into the freezer and carried to the rack that held the framed screens; within 5 minutes even the heaviest garments appeared to be frozen. The racks of screens were covered with black plastic to exclude light during storage. Some wind chill was apparent even within the covered screen racks, and apparent slow drying has occurred during storage.
The trunk contained 8 woolenlike long underwear shirts or pants; 2 pairs of men's pants; 53 linenlike shirts, collars, chemises, gowns, long underwear (men's and women's), and handkerchiefs; 11 sashes of varieties of construction; 15 pairs of stockings or socks; 3 silklike scarves; 2 pairs of gloves; 1 leather jacket; 1 leather vest; 4 other vests; 2 smoking jackets or robes; and 1 towel. Other artifacts in the trunk that were frozen included a newspaper, the baffle material, and the lining of the trunk. Large fragments of the lining fell free from the trunk interior, so some were removed in the wet state and stored wrapped in plastic in a refrigerator until the drying experiments were conducted. A database of the artifact types, samples taken, and descriptions has been constructed.
4 DRYING EXPERIMENTS
In drying waterlogged textiles, consideration must be given to the possibility that the fibers could collapse on removal of the water they contain. An ideal drying method would preserve the fiber and fabric structure while allowing the textile to be stored in ambient conditions in a museum. To determine the best drying method, samples of wet trunk lining were treated in the following ways:
- Air dried. A sample of trunk lining approximately 1 � .6 cm was allowed to dry in a desiccator in the laboratory.
- Ethanol dehydration. A sample of trunk lining approximately 1 � .6 cm was treated by immersing it in gradually increasing concentrations of ethanol/distilled water. The sample was placed in baths of 50/50, 60/40, and 70/30 ethanol/water for 30 minutes each, then in baths of 80/20, 90/10, and 95/5 ethanol/water for 60 minutes, and finally in two 100% ethanol rinses. After the second 100% ethanol rinse, the sample was allowed to dry in ambient conditions in the laboratory, while being protected from dust, and then was placed into a desiccator. The goal of ethanol dehydration was to dry the waterlogged material by replacing the water with ethanol and then evaporating the ethanol. In this manner, the collapse of fibers on removal of the fluids they contain could be eliminated.
- Critical-point drying. A sample of trunk lining approximately .6 � .6 cm was treated in the same steps as the ethanol dehydration, except that on immersion in the second 100% ethanol rinse, the ethanol-soaked sample was placed in a Denton Vacuum Critical Point Dryer, Model DCP-1. Critical-point drying was accomplished using liquid carbon dioxide as an exchange fluid. After drying, the sample was placed in a desiccator. The critical point-drying technique allows a wet sample to be dried completely with minimal disruption of structure caused by the surface tension effect of water. The critical temperature—the temperature above which a gas cannot be liquified by additional pressure—and the critical pressure—the pressure under which a substance may exist in equilibrium as a gas with a liquid at critical temperature—are both met in critical-point drying. As a result, there is no phase boundary between the liquid and gas phases. Since water has such a high critical temperature (approximately 375�C) and critical pressure (3,212 psi), it is replaced with a more convenient fluid, CO2, which has a critical temperature of 31�C and critical pressure of 1,072 psi. However, since CO2 and water are not miscible, the water is replaced with ethanol by introducing the sample into ethanol/water mixtures containing increasing concentrations of ethanol and decreasing concentrations of water, ending in 100% ethanol. Ethanol is miscible with both water and CO2, so the ethanol-wetted sample is placed in a pressure chamber and flushed with liquid carbon dioxide to replace all of the ethanol. The temperature is then raised to just above 31�C, the critical point, and the gaseous carbon dioxide is slowly vented to avoid distorting the sample.
- Vacuum freeze drying. A trunk lining sample approximately .6 � .6 cm was placed in a rapid freezer (−19�F) while still wet. The frozen sample piece was then placed in a Labconco Freeze Dry 5 Model 75050 freeze dryer unit and processed at a pressure of 0.1 μm (1 � 10−4 Torr [mm of Hg]). The dried sample was then placed in a desiccator. Freeze drying is often used in specimen preparation. To avoid the surface tension distortion effects of liquid water, the sample is frozen and a vacuum is applied. Water is removed by sublimation, avoiding the liquid phase.
- Frozen and slowly dried. A sample approximately .6 � .6 cm in size was removed from a larger section of trunk lining that had been kept at −19�F since the salvage operation in November 1990. With the approximate 5 mph air current in the freezer, the effective temperature was approximately −26�F. The trunk lining was dry when it was removed in January 1991. Due to the low humidity of the freezer, the ice contained in the fabric had sublimed. No method had been established for measuring the drying rate in the freezer; the fabric is labeled “slowly dried” because it dried more slowly than the vacuum-dehydrated sample.
A man's handkerchief that had been slowly dried in the freezer was also selected for examination by scanning electron microscopy. Two samples less than 1 cm square were studied.
Scanning electron microscopy and energy-dispersive x-ray analysis (EDS) were carried out on each of the test specimens, using a Jeol JSM-820 scanning electron microscope with Tracor Northern TN-5501 EDS unit. The sample was mounted with colloidal graphite on a spectroscopically pure carbon planchette. The entire area of the sample was examined to assess its overall condition. Scanning electron micrographs of representative areas of each of the samples were taken at 25, 250, and 500 x magnification. No statistical analysis was undertaken; the results of the drying treatments were evaluated visually.
5 RESULTS AND DISCUSSION
The trunk lining is a coarse, plain-woven fabric with a yarn count of 44 � 51 yarns per in. Examination of trunk lining fibers by light microscopy confirms their identification as linen. The fiber structures display extensive microbiological damage. EDS analyses of the fibers in each of the fabrics indicated a wide array of elemental compositions reflecting contamination by metal corrosion and by products of biological degradation as well as permeation of the textiles with sea water. Significant amounts of iron and/or copper and/or sulfur as well as smaller amounts of calcium, phosphorus, silicon, aluminum, and magnesium were observed in the textiles.
The fabric of the air-dried trunk lining (fig. 1) shows some surface tangling of fibers, but the fabric structure is still apparent. At higher magnification (fig. 2) the fibers appear round, with some eaten-away areas due to microbial damage. The fibers range in size from 10 to 20 μm in diameter. Foamy deposits of contaminants were observed in some areas of the fiber surfaces, but they do not obscure the fabric structure.
Fig. 1.
Scanning electron micrograph of air-dried trunk lining (15 x)
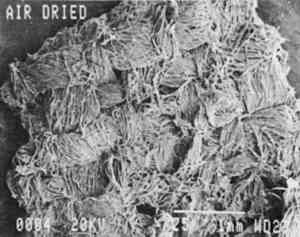 |
Fig. 2.
Scanning electron micrograph of air-dried trunk lining (300 x)
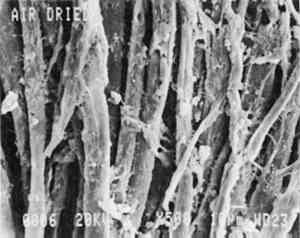 |
Fabric dried from ethanol also displayed some surface tangling. In addition, the fibers appear flattened and stuck together (fig. 3). The fabric structure is not as clear as that of the air-dried sample. Although the samples were taken from the same trunk lining, it is possible that different sections of fabric experienced different stresses during the performance life of the fabric. Yarns that experienced more compressive force will be flattened due to that force. However, if the lack of definition of the fabric structure is due to the drying process, the ethanol dehydration is a much less desirable method.
Fig. 3.
Scanning electron micrograph of trunk lining dried from ethanol (15 x)
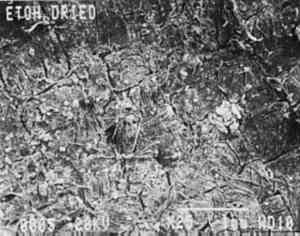 |
Fabric dried by critical point procedures appears more clean and less entangled than the previous two samples (fig. 4). Although yarn crowns display flattening in some areas, the fabric structure is readily apparent. There are some small areas of crusty deposit. It is possible that the solutions used in the drying process may have served as cleaning agents, removing some of the contamination as the fabrics were immersed in them. At higher magnification (fig. 5), the air-dried and critical point-dried fibers appear to be comparable in clarity, size, and quantity of deposits overall.
Fig. 4.
Scanning electron micrograph of trunk lining dried by critical-point procedures (15 x)
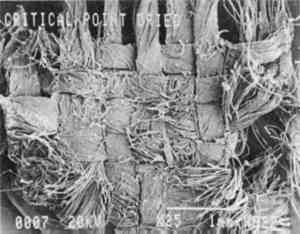 |
Fig. 5.
Scanning electron micrograph of trunk lining dried by critical-point procedures (300 x)
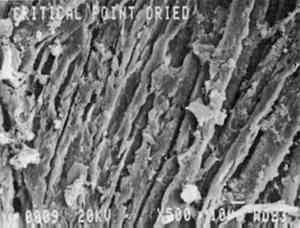 |
Most damaging to the appearance of the fiber and fabric structure is the vacuum freeze-drying treatment. As can be seen in figure 6, the surface of the yarns and fibers are covered with a crust of amorphous material that obscures their appearance so that the characteristic structures of the linen fabric cannot be seen. The overall fabric appearance shows considerably more alteration than that seen in the air dried and critical point-dried samples. The cause of this great extent of encrustation lies either in the freezing of the water or in the application of a vacuum in this treatment. In freezing water, the formation of the water crystals may have pushed the contaminants out of the fiber onto the front of the ice structures being formed. On the other hand, the vacuum may have drawn the contaminants to the surface of the fibers.
Fig. 6.
Scanning electron micrograph of trunk lining dried by vacuum freeze drying (15 x)
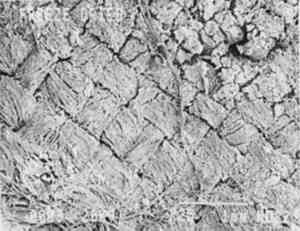 |
Comparison of the vacuum freeze-dried sample to the sample that was slowly dried in the freezer (fig. 7) reveals that the latter treatment did not produce large amorphous encrustations. Although some fiber entanglement is seen, the fabric structure is readily apparent. The fiber surfaces of frozen and slowly dried fibers are clean and display few encrustations. They are comparable in appearance to both the critical point-dried and air-dried material. It seems apparent, then, that the vacuum applied in the vacuum freeze-drying procedure is sufficient to draw the contaminants out of the fibers and create deleterious results.
Fig. 7.
Scanning electron micrograph of trunk lining slowly dried in the freezer (15 x)
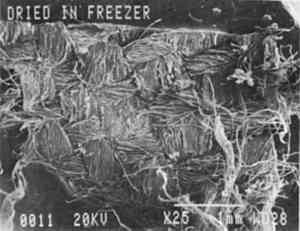 |
A handkerchief that had been dried slowly in the freezer was examined for comparative purposes. The fabric structure of the handkerchief is readily apparent (fig. 8). This is a fine plain-woven fabric with a count of 110 � 119 yarns per in. Although there are some entangled fibers, the appearance is not occluded by deposits. At higher magnification (fig. 9), some wispy deposits are noted, but the fibers can be distinguished. In this case, the structure is clear after slow drying in the freezer.
Fig. 8.
Scanning electron micrograph of men's handkerchief slowly dried in the freezer (15 x)
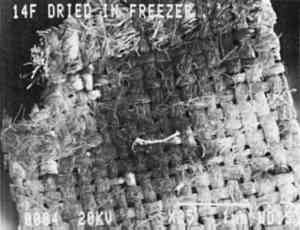 |
Fig. 9.
Scanning electron micrograph of men's handkerchief slowly dried in the freezer (300 x)
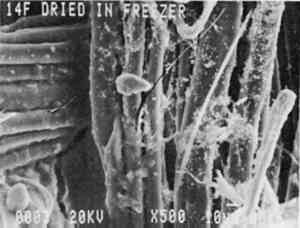 |
The satisfactory visual results obtained through slow drying in the freezer support that choice as appropriate in the drying of waterlogged textiles. While air drying is often the method of choice simply because the conservator's laboratory is not equipped for anything else, if there is question concerning the identity and condition of the fibers, the effects of air drying would be unknown. Freezing appears to be a preferred choice when there is an immediate need to preserve textile items from deterioration, as would be the case when there is a large quantity of waterlogged material and the individual pieces cannot be assessed and conserved immediately. The waterlogged material can be dried with no apparent damage to fiber structure; degradation by any mechanism is effectively eliminated.
Beyond air drying, slow drying in a freezer compares favorably with the other techniques investigated. Freezers are also widely available and cost less than some of the equipment needed for other drying methods. In the event of disasters with water, emergency freezing facilities have been devised. Koesterer and Geating (1976) report that a walk-in thermal chamber designed for spacecraft testing was used to vacuum freeze dry books that had been soaked with water in the quenching of a library fire. Access to a freezer facility at the Ohio State University allowed the rapid freezing of the large quantity of textiles salvaged from the trunk. The cost of renting freezer space was minimal; the greatest expense was the construction of framed screens to support the garments. Local universities or food service facilities could provide access to walk-in freezers and refrigerators. These freezers are large enough to accomodate the large screens.
Most freeze-dry units used in research are small because they rely on vacuum pumps to obtain sufficiently low pressure while maintaining sufficiently low temperature. Larger units are available with larger more costly vacuum pumps. Although the equipment can be expensive, operation is inexpensive, requiring only the cost of the cooling fluid to maintain the low chamber temperatures.
Due to the process of fluid exchange in the critical-point dryer, the maximum size of commercially available equipment is approximately 0.5 ft3 or smaller, most critical-point dryers are much smaller. Units could be made to larger dimensions, but with concomitant increasing cost since the chamber must withstand pressures of more than 1,200 psi. As mentioned, critical-point drying was examined for experimental purposes in this work to study differences in the structure of fibers dried by different methods. While critical-point drying is not feasible for large textile items, the comparable appearance of fibers after critical-point drying and after slow drying in the freezer supports the suitability of slow drying for waterlogged materials.
Ethanol dehydration may be an attractive choice because it can be conducted in a standard laboratory with appropriate ventilation conditions and because the visual results obtained are satisfactory. However, it is less desirable because it may incur some change in the chemistry of the materials being treated. Freezing textiles in their natural state results in no chemical alteration of the fibers, whereas ethanol dehydration may dry fats or oils from some of the textiles.
6 CONCLUSIONS
The emergency situation presented by a trunk full of waterlogged garments threatened by deterioration led to the prescription of a plan that entailed unrolling and arranging the textiles underwater while in the plasticized state and then freezing them rapidly. An examination of different methods of drying linen trunk lining textiles suggested that slow drying of frozen textiles appeared to be a less disruptive method of drying and preservation than vacuum freeze drying. Vacuum freeze drying was more disruptive in its effects than air drying, ethanol dehydration, critical-point drying, or slow drying in the frozen state. Although ethanol dehydration and critical-point drying gave satisfactory visual results, these methods are less desirable because chemical change in the material is possible due to the solvents involved. In addition, the use of critical-point drying is limited by commercially available chamber sizes. Drying waterlogged material by critical-point methods, did show, however, that fiber structure yielded by this method was comparable to that obtained by slow drying.
This paper suggests that a freezer facility may be accessible to the conservator through a local university or food service facility at minimal cost. Those involved with disaster planning may consider the implications of these results with respect to treatment of quantities of waterlogged textiles, particularly those that may contain many contaminants but cannot receive immediate conservation treatment.
The work reported was limited to visual evaluation of the suitability of different drying methods. Perhaps some other factors are involved in the appearance of fibers examined. There may have been some differential flat abrasion of the trunk lining not related to the drying method that resulted in a different appearance from one location to another. Additional work with waterlogged marine textiles will assess this possibility. Future papers will also deal with the identification and charcterization of fibers from textiles recovered from the marine environment and with the experiments in conservation treatment conducted for the stabilization of marine textiles.
ACKNOWLEDGEMENTS
The support of the Columbus-America Discovery Group in the conduct of this research is gratefully acknowledged. Salaries and research support were provided in part by state and federal funds appropriated to the Ohio Agricultural Research and Development Center, Ohio State University (manuscript no. 98-91).
REFERENCES
Bengtsson, S.1975. Preservation of the Wasa sails. In Conservation in archaeology and the applied arts. London: International Institute for Conservation. 33–35.
Conrad, J.1991. Final voyage. American History Illustrated26(1):58–65, 72.
Conrad, J., ed.1988. Story of an American tragedy. Columbus, Ohio: Columbus-America Discovery Group.
David, A.1981. Freeze drying leather with glycerol. Museum Journal81:103–04.
Ganiaris, H., S.Keene, and K.Starling. 1982. A comparison of some treatments for excavated leather. Conservator6:12–23.
Goodway, M.1987. Fiber identification in practice. Journal of the American Institute for Conservation26:27–44.
Grattan, D. W.1989. Treatment of waterlogged wood. In Wet site archaeology, ed.B. A.Purdy. Caldwell, N.J.: Telford Press. 237–54.
Grosso, G. H.1975. Wood, textile, and leather conservation techniques for the archaeologist. Northwest Anthropological Research Notes9(1):180–97.
Harpers Ferry Regional Textile Group. 1986. Textile treatments revisited. Washington, D.C.: Harpers Ferry Regional Textile Group.
Herdendorf, C. E.1991. Discovery in an alien environment. American History Illustrated26(1):66–71.
Jakes, K. A., and Angel, A.1989. The determination of elemental distribution in ancient fibers. In Archaeological chemistry IV, ed.R. O.Allen. Advances in Chemistry series 220. Washington, D.C.: American Chemical Society. 451–64.
Jakes, K. A., and Sibley, L. R.1989. Evaluation of a partially mineralized fabric from Etowah. In Archaeometry; Proceedings of the 25th International Symposium. ed.Y.Maniatis. New York: Elsevier. 237–44.
King, M. E.1978. Analytical methods and prehistoric textiles. American Antiquity43(January):89–96.
Koesterer, M. G., and Geating, J. A.1976. Restoring water-soaked papers and textiles: Applying freeze-drying methods to books and art objects. Technology and Conservation1:20–22.
Lore, D.1990. Gold ship provides bonus of clothing. Columbus Dispatch, November 11, 6C.
Mayhew, F.1990. Private communication.
McCawley, J. C.1977. Waterlogged artifacts: The challenge to conservation. Journal of the Canadian Conservation Institute2:17–26.
Mellor, J. D.1978. Fundamentals of freeze drying. London: Academic Press. 330.
Morris, K., and B. L.Seifert. 1978. Conservation of leather and textiles from the Defence. Journal of the American Institute for Conservation18:33–43.
Norton, R. E.1990. Conservation of artifacts made from plant materials. In Conservation of artifacts made from plant materials, ed.M. E.Florian, D. P.Kronkright, and R. E.Norton. Los Angeles: J. Paul Getty Trust.
Peacock, E. E.1990. Freeze drying archaeological textiles: The need for basic research. In Archaeological TextilesOccasional Papers 10, ed.S. A.O'Connor and M. M.Brooks. London: United Kingdom Institute for Conservation. 22–30.
Pearson, C., ed.1987. Conservation of marine archaeological objects. New York: Butterworths.
Ringle, K.1990. Salvaging the gold of history. Washington Post, November 29,. D1, D8
Rosenquist, A. M.1975. Experiments on the conservation of waterlogged wood and leather by freeze-drying. In Problems in the conservation of waterlogged wood, ed.W. D.Oddy. London: National Maritime Museum. 9–23.
Ryder, M. L., and T.Gabra-Sanders. 1985. The application of microscopy to textile history. Textile History16(2):123–40.
Schaffer, E.1981. Fiber identification in ethnological textile artifacts. Studies in Conservation26:119–29.
AUTHOR INFORMATION
KATHRYN A. JAKES holds a Ph.D. in textile and polymer science from Clemson University. She is a professor in textiles at Ohio State University, and she conducts research in the identification and characterization of degraded polymeric materials. Much of her work deals with historic and archaeological textile materials. Address: Department of Textiles and Clothing, Ohio State University, 262 Campbell Hall, 1787 Neil Ave., Columbus, Ohio 43210-1295.
JOHN C. MITCHELL holds a bachelor's degree from Ohio State University, where he is an instructor in SEM techniques and the manager of the Department of Geology's Scanning Electron Microscopy Facility. Address: Scanning Electron Microscopy Laboratory, Department of Geological Sciences, 002 Orton Hall, 155 South Oval Mall, Columbus, Ohio 43216-1295.
Section Index |