ASSESSMENT OF DETERIORATION IN ARCHAEOLOGICAL WOOD FROM ANCIENT EGYPT
ROBERT A. BLANCHETTE, JOHN E. HAIGHT, ROBERT J. KOESTLER, PAMELA B. HATCHFIELD, & DORTHEA ARNOLD
3 RESULTS AND DISCUSSION
3.1 WOOD ULTRASTRUCTURE
The patterns of wood deterioration were determined by studying transverse sections of degraded woods with transmission electron microscopy. These methods allowed high magnification observations to be made of the woody cell wall with good resolution. Sound wood from modern samples are presented to familiarize the reader with the ultrastructure of unaltered woods (fig. 1). Wood from angiosperms (hardwoods such as maple and oak) or gymnosperms (conifers such as pine and spruce) are composed of different types of cells(fig. 1a–b). Angiosperms have vessels and fibers whereas gymnosperms have tracheids. Both contain various types of ray parenchyma cells. In some angiosperms such as boxwood, the wood contains relatively small vessels and thick-walled fiber-tracheids (fig. 1c). Woody cell walls have distinct morphological regions that can easily be differentiated. A dark region between cells is the compound middle lamella (fig. 1a–c). This area contains large concentrations of lignin, and since KMnO4 (a compound that reacts with lignin resulting in an electron dense stain) was used to fix the wood, this area appears most electron dense. The secondary wall consists of three layers commonly referred to as the S1, S2, and S3(fig. 1a–c). The S2 layer of fibers and tracheids contains the greatest amounts of cellulose along with some lignin and is the largest layer. In fiber-tracheids of boxwood, the S2 layer is exceedingly thick (fig. 1c).
Fig. 1.
Transverse sections of (A) sound modern maple (Acer); (B) spruce (Picea); and (C) boxwood (Buxus), showing various morphological regions of woody cell walls. The dark, electron dense regions between cells is the compound middle lamellae (ML), and the secondary wall has S1, S2, and S3 layers. (A) Maple is an angiosperm with vessels (V) and fibers (F) evident in the wood. The secondary wall layers of vessels are approximately equal in size, whereas fiber walls have a large S2 region. (B) Tracheids of the gymnosperm, spruce, showing middle lamellae and secondary walls. (C) Wood from the angiosperm, boxwood, is unusual in that it contains small vessel elements (not shown in figure) and fiber-tracheids. The fiber-tracheids have an exceedingly thick S2 layer of the secondary wall. Bar = 5 μm
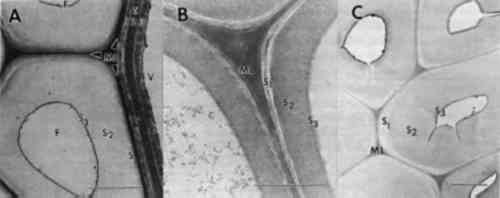 |
3.2 GENERAL OBSERVATIONS OF WOODEN CULTURAL PROPERTIES
Objects made from wood are well represented in museum collections of ancient Egyptian art. Statues, coffins, furniture, and other cultural properties have been found in ancient tombs in remarkably good condition considering they have been buried for thousands of years. Deterioration, however, is common among these objects, and it is rare to find wooden cultural properties that are totally free of decay. In many collections, the true condition of artifacts may be masked by materials such as wax initially used to facilitate their removal from burial environments, or by early restoration techniques designed to improve the aesthetic appearance of the object for display purposes. Only limited study has been initiated on the effects of some of these treatments on the condition of ancient woods (Hatchfield and Koestler 1987). Nor has the effect of various restoration treatments on the fragile condition of the altered, deteriorated wood been investigated. Extensive restoration, completed more than 50 years ago, is shown in a photograph of the statue of the scribe of Mitry (MMA 26.2.4) taken during the restoration process (fig. 2a). The completed work shows little evidence of decay that was so prevalent in the object. In comparison, the decayed statue of Mitry's wife (MMA 26.2.5), found in the same tomb with MMA 26.2.4 and not restored, reveals the true condition of the wood (fig. 2b). The unaltered statue allows an evaluation of the events that influenced the deterioration and provides the opportunity to learn more about the aging process of wood, microbial interactions, and the past physical environment of the tomb. Many objects we examined had advanced decay, whereas others had decay that was restricted to the surface or found in localized areas of the wood (fig. 3). The deteriorated remains of gesso and paint are also present on many objects (fig. 2a–b).
Fig. 2.
Cultural properties from ancient Egyptian tombs showing extensive deterioration. (A) Statue of the scribe of Mitry, V Dynasty (2340 B.C.) from Saqqara, Metropolitan Museum of Art, New york (MMA 26.2.4). Large zones of decayed wood were removed and filled with plaster during restoration work completed more than 50 years ago. (B) Statue of the wife of Mitry (MMA 26.2.5) excavated from the same tomb. Deterioration is severe, especially at the lower portions of the statue that were in contact with the tomb floor.
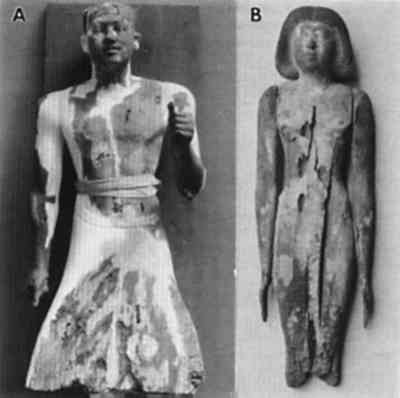 |
Fig. 3.
(right) Deterioration in objects from ancient Egyptian tombs. (A) and (B) Mask from a coffin lid, XVIII Dynasty (1570-1293 B.C.) from Abydos Tomb D115, currently in the Museum of Fine Arts, Boston (MFA 01.7431). Front view is shown in (A) with deteriorated gesso and paint. The back side of the mask (B) exhibits advanced stages of decay throughout the wood. (C) Outer coffin of Gem-hap (MFA 29.1860) from Giza (664-525 B.C.), showing deterioration of coffin surface. (D) Statue of a girl (MFA 13.3464), V Dynasty (2524-2400 B.C.) from Giza, with advanced deterioration that has led to severe surface defects.
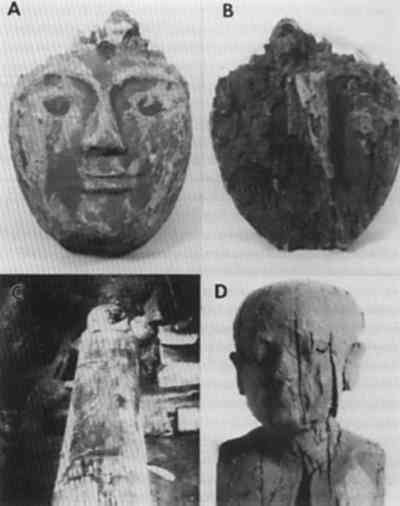 |
3.3 NONBIOLOGICAL DETERIORATION
Samples of wood taken from the outer surfaces of many objects were found to have wood cells that displayed numerous cracks and fractures within cell wall layers. Separations were frequent within the compound middle lamella region (fig. 4). This electron dense region between cells appeared granular and lacked integrity. In some cells with alterations, the middle lamella was extensively fragmented, and secondary walls were detached from adjacent cells (fig. 4b). Observations of detached cells showed remnants of the middle lamellae adhering to the S1 region of the secondary wall. Cracks and separations were also evident in secondary wall regions. The separations often formed large voids in the wall or occurred in a parallel series of small separations within the S2 layer. Extraneous materials were frequently observed in cell lumina (fig. 4a–b).
Fig. 4.
(below) Transmission electron micrographs of transverse sections from the mask from a coffin lid (A) and (C) (MFA 01.7431, see fig. 3A–B) and bed leg (B) (MFA 13.2.781), showing a nonbiological form of deterioration that affected the exterior portions of the wood. (A) and (B) Delaminated wood cells with cracks and fissures in the middle lamella (ML) region. Large voids are present in cell corners (CC). Cell walls appear swollen and have separations within the secondary wall layers (S). Parallel series of separations appear within the expanded S2 layer (arrows). Extraneous meterial is evident, partially filling the cell lumina (∗). (C) Affected cells detach from adjacent cells with remnants of degraded middle lamellae (ML) adhering to the secondary walls (S) (arrowheads) Bar = 5 μm
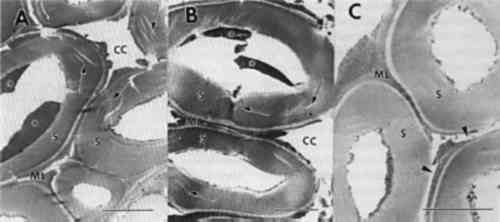 |
Previous investigators who examined the ultrastructure of wooden objects from ancient Egypt reported checks and fractures occurring in otherwise sound wood cells (Borgin et al. 1975; Nilsson and Daniel 1990). A general weakening of the wood structure was apparent, causing delamination and loosening of wall layers. The fissures and other alterations were thought to be exaggerated due to the preparation procedures used for ultrastructural observations (Borgin et al. 1975; Nilsson and Daniel 1990).
From the objects that we examined, nine showed some evidence of cell wall weakening with delaminations in the secondary wall and fissures occurring in the middle lamella regions. No evidence of microorganisms was found in these altered cells. The degradation patterns, when compared to the various forms of decay that have been evaluated, most closely resembled alterations associated with a delignification process. This type of damage may occur from degradation by white rot fungi that selectively remove lignin (Blanchette et al. 1990; Blanchette 1991; Eriksson et al. 1990), photodegradation by ultraviolet light (Chang et al. 1982; Kuo and Hu 1991), or other nonbiological forms of deterioration (Blanchette et al. 1991a; Kass et al. 1970; Parameswaran 1981).
Decay by white rot fungi did not occur since no evidence of fungal hyphae or other characteristics of wood decay by white-rotters was evident. In addition, the conditions of these burial sites containing limestone and alkaline pH conditions are not conducive to fungi in the class Basidiomycetes that cause a white rot (Blanchette et al. 1990). It is unlikely that these objects were exposed to ultraviolet radiation in amounts that would induce photo-oxidation processes. The most plausible explanation for this deterioration appears to be chemical reactions occurring when wood is in contact with limestone, gesso, or various salts over long periods of time. Unfortunately, little is known about the nonbiological degradative processes that take place when wood surfaces are in contact with alkaline substances for thousands of years. Moisture, crucial to initiating reactions, was apparently intermittently present in many tombs. The migration of salts into wood also occurred during burial. Elemental analyses of two samples of wood, one from a limestone burial chamber and the other a beam used in transportation, showed high concentrations of sodium and calcium within the wood (table 2). The exceedingly high concentrations of sodium and other salts found in the beam demonstrate the levels that accumulate in wood when it is buried in contact with gypsum plaster.
When high concentrations of alkali are used in the pulping process of wood for papermaking, delignification results. The alkali treatment of wood combined with heat causes a dissolution of hemicellulose and lignin from wood, resulting in a cellulose-rich pulp. Wood in contact with low concentrations of alkaline substances may also be affected. Surface deterioration has been found in wood that was in contact with potash for 40 years. The degradation caused a delamination of wood cells and a general dissolution of lignin. The cells appeared partially swollen and sustained cracks and fissures within the secondary wall. Potassium chloride crystals were deposited on surfaces between cells and within cell walls. In areas where potassium chloride crystals accumulated, a total hydrolysis of wall material was evident (Parameswaran 1981). A similar type of deterioration has been reported previously where alkali substances were in contact with wood for several years within a historic building (Blanchette et al. 1991b).
Surface deterioration identified in the ancient Egyptian wood examined mimics the chemical deterioration patterns observed when high concentrations of alkaline substances come in contact with wood. Limestone or gesso may serve to initiate this nonbiological form of degradation when moisture was present within the tombs. Although concentrations of alkaline substances may be low, exposure for exceedingly long periods of time may have significant effects. High salt concentrations were present in two samples used for elemental analyses (table 2). These accumulations suggest that moisture from flooding, heavy rains, or other sources percolated down into the burial sites, causing various salts to migrate into the wood. The presence of sodium chloride frequently occurs within limestone in the Nile Valley, and physicochemical conditions of Egyptian archaeological sites have previously shown that long-term chemical reactions promoted by the presence of both sodium chloride and moisture affect plaster and painted murals within tombs (Burns et al. 1989).
The corrosive action of elevated salt concentrations also appears to contribute to wood deterioration (Wilkins and Simpson 1988). These high concentrations of salt pose serious concerns for conservators and may result in water absorption if humidity and temperature are not controlled. Without proper safeguards, surface deterioration may continue, albeit slowly, within excavated wood if moisture is present. Additional study of interactions among wood, moisture, sodium chloride, ancient gypsum-base plasters (and impurities) as well as limestone burial sites needs to be considered to understand fully the process of this nonbiological deterioration.
3.4 BIOLOGICAL DEGRADATION
In addition to areas of limited surface deterioration, many objects had extensive degradation throughout large areas of the wood (figs. 1–2). Macroscopically, the degraded wood appeared brown with little integrity remaining. Sections made from the various degraded objects showed that cell walls were decayed by fungi. Two distinct forms of decay were evident. Some objects had decay with characteristics of soft rot, whereas others were decayed by brown rot fungi.
3.4.1 SOFT ROT
Decay by soft rot fungi can be identified by the presence of longitudinal cavities that occur within the secondary wall layers of wood cells (Blanchette et al. 1990; Eriksson et al. 1990). In transverse sections, the cavities appear as round holes within the cell walls. In some of the wood samples examined, a typical soft rot form of decay was evident. Numerous holes were observed within the secondary walls, with remnants of fungal hyphae present within the cavities (fig. 5a–b). An electron dense area was observed around the cavities within the walls (fig. 5c). Cells with relatively few small diameter cavities represented early stages of decay (fig. 5a), whereas cells with numerous holes throughout the secondary wall represented late stages (fig. 5b–d). Holes within secondary walls often coalesced, forming large voids (fig. 5d). The middle lamella was occasionally disrupted. Cells with advanced soft rot lost much of their integrity, and the wood was extremely weak. Soft rot, when it has advanced to this stage of degradation, has removed most if not all of the woods' original strength properties (Hoffmeyer 1976).
Fig. 5.
Transmission electron micrographs showing soft rot cavities within transverse sections of cell walls from objects in the Museum of Fine Arts, Boston: (A) MFA 21.899; (B) MFA 01.7431; (C) and (D) MFA 21.897; and in the Metropolitan Museum of Art: (E) and (F) MMA 27.9.5 (A) Incipient stages of decay showing small cavities within the secondary wall layers (S) (arrows). (B) Large cavities (arrows) within the secondary wall (S), with remnants of fungal hyphae located within the cavities. (C) Soft rot cavities within the cell wall have a large central void with fungal hyphae (H) present. The area of the secondary wall around the degraded hole is extremely electron dense (arrowheads). (D) Advance stages of decay, with numerous cavities throughout secondary wall layers. Cavities coalesce, forming large holes within the cell wall that reduce wood strength and integrity. Middle lamellae regions remain relatively free of attack but often fragment due to the lack of intact secondary wall material. (E) An unusual form of soft rot with cavities in the interior portions of the woody cells. The thick wall layer within cells resembles the gelatinous layer (G) found in tension wood. Cavities (arrowheads) are restricted to the reduced S1 and S2 regions of the cell wall and appear elongated. (F) Cells with advanced decay have eroded S1 and S2 layers (arrows) and fragmented middle lamellae. The thick gelatinous layer (G) is not degraded except for small diameter cavities that resemble hyphal penetration sites. Bar = 5 μm
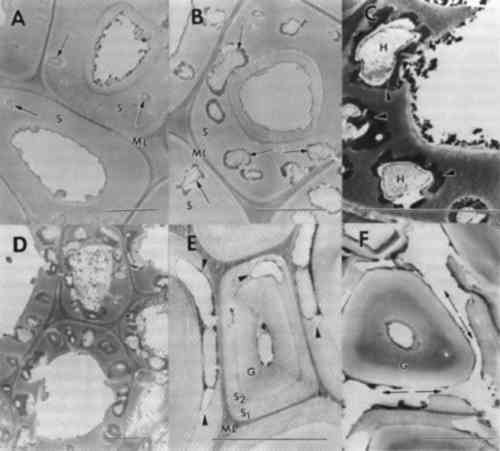 |
An unusual form of soft rot was evident in one object (MMA 27.9.5). Cavities did not form in the thick layer of the secondary wall but were located within the innermost regions of this layer (fig. 5e–f). The thick secondary wall occasionally had small penetration holes (fig. 5f), but even after advanced soft rot, this part of the cell wall resisted decay. These cells appear to have characteristics common to tension wood. Tension wood is formed on the upper surface of leaning hardwood trees but can also occur in nonleaning tropical trees (Panshin and de Zeeuw 1970). Tension wood cells have a thick secondary wall layer that has been termed a gelatinous layer. The microfibrillar orientation of cellulose and the chemical composition of this layer are different from normal wood. A more crystalline form of cellulose has also been identified from the gelatinous layer (Wardrop and Dadswell 1955). The cavities within the cell wall were located within the S2 and S1 regions and were more elongate than typical soft rot cavities. Numerous coalescing cavities resulted in disrupted middle lamellae and detached remnants of cell walls (fig. 5f).
Soft rot fungi are most frequently associated with wood in contact with excessive moisture, conditions that exclude other more aggressive wood destroying fungi. Recently, it has been shown that soft rot fungi are prevalent in wood with low moisture or in environments with high pH (Blanchette et al. 1990; Blanchette and Simpson 1992). In these unusual environments, soft rot fungi progressively attacked the wood, causing a slow degradation whenever minimum conditions for growth were met. The extent of decay can be substantial over time, as demonstrated in a study of wood from Tumulus MM in Gordion, Turkey, where enormous logs of Cedrus and Juniperus (trees with significant decay resistance [Scheffer and Cowling 1966]) were decayed extensively by soft rot fungi over a 2,500-year period under very limiting moisture conditions (Blanchette et al. 1991b; Blanchette and Simpson 1992).
3.4.2 BROWN ROT
Not all objects with extensive degradation were decayed by soft rot fungi. Observations made from sections of several other objects were found to be degraded by brown rot fungi. Wood cell walls from the decayed samples sustained a general loss of wood integrity, causing secondary walls and middle lamellae to appear swollen, porous, and distorted (fig. 6a–c). In cells with advanced decay, secondary cell walls disintegrated into a granular mass of residual wall material (fig. 6d). The cell corner regions of the middle lamellae remained relatively intact, but the zone of middle lamella between cells was severely disrupted (fig. 6c–d). In many areas of the wood, the cell walls fragmented into minute particles that filled cell lumina. Remnants of fungal hyphae were present in all woods examined.
Fig. 6.
Transmission electron micrographs of transverse sections showing different stages of brown rot in samples from the Museum of Fine Arts, Boston, and the Metropolitan Museum of Art: (A) MFA 02.813; (B) MMA 12.187.52; (C) MMA 26.2.4; (D) MMA 26.2.5; (E) MMA 32.1.113; (F) MFA 29.1860. (A) Incipient stages of decay in earlywood cells with some loss of integrity as evident in cell walls that bend and have lost some of their original shape (arrowheads). (B) A more advanced stage of brown rot, with secondary walls (S) exhibiting a swollen appearance and convoluted middle lamellae (ML) with reduced electron density. (C) and (D) Secondary walls (S) have become extended and appear to have a granular consistency. Cell walls have altered shapes, with areas of the middle lamellae between cells distorted and disrupted. Cell corner regions of some cells remain but have a diffuse, swollen appearance. The secondary wall layers can still be identified with remnants of fungal hypha in cell lumina (arrows). (E) In advanced stages of decay the secondary wall layers have been disrupted, and residual secondary wall substances (S, arrows) are dispersed. A residual middle lamellae can be seen among degraded particulate matter. (F) Severe deterioration is evident in totally disrupted cells. Degraded secondary walls (S) and middle lamella (ML) have fragmented into minute dustlike particles. Bar = 5 μm
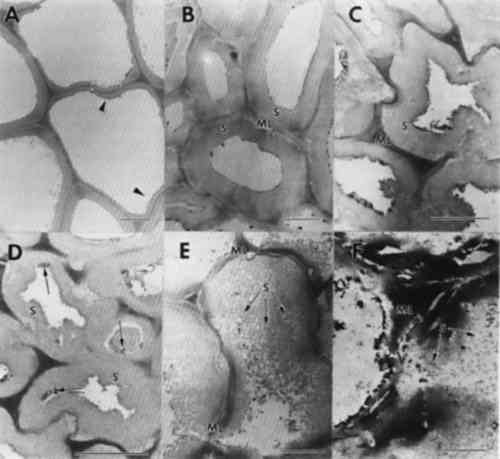 |
Brown rot fungi cause a diffuse depolymerization of cellulose in wood, resulting in significant strength losses during incipient stages of decay (Blanchette et al. 1990). As decay progresses, polysaccharides are degraded, leaving residual wall material that consists primarily of modified lignin. The cell walls of brown-rotted wood have poor integrity, and wood can be easily crushed to a dustlike powder. This type of decay raises serious concerns for removing the object from the burial site without damage and for identifying proper conservation methods needed to stabilize the wood. Earlier in the century, archaeologists and conservators had few means of assessing damage to artifacts other than visual examination, and used the best materials and technology available in their efforts to preserve them. Opinions were, as they are today, strong about the expected appearance of objects for exhibition. A beautiful appearance indicated a sound object. However, we know that treatments such as those shown in figure 2 destroy much of the original wood and introduce the possibility of additional physical and chemical alterations that could have detrimental effects. Although advances in conservation practices for archaeological wood have been made in recent years (Barclay 1981; Schniewind 1990), an appropriate method of treating deteriorated wood without possible adverse affects has not been identified. Knowledge of the subcellular condition of the wood and characteristic physical and chemical properties is essential for developing specific treatments that will ensure long-term conservation and protection of these important cultural properties. Research into the possible long-term effects of gypsum, polyfillers, or other conservation products used in the conservation of wood is especially warranted. Although damage as a result of relatively short-term contact between archaeological wood and alkaline fill materials has not been identified, alternatives should be considered, such as glass microballoons in acrylic resins (Hatchfield 1986). This substitution avoids exposing archaeological wood to both excessive moisture and alkaline substances, which could promote accelerated deterioration in localized areas.
Wood decayed by brown rot fungi was frequently encountered in the archaeological wood examined from ancient Egypt. Moisture is essential for brown rot to occur and had to have been present in sufficient quantities for decay to progress to the advanced stages observed. Excavation photos indicate that the most severe decay was located in wooden objects in contact with the tomb floor or along walls (Zayed 1956). These sites of moisture accumulation may have occurred relatively soon after the tomb was closed and when propagules of brown rot fungi were still viable. Evidence of termites and other insects in some burial sites suggests the possibility that decay fungi could be introduced at later times by insects that entered the tomb and disseminated the brown rot fungi.
3.4.3 DETERIORATION PATTERNS MASKED BY TREATMENT
Not all deterioration observed in wood from museum collections can be attributed to biotic or abiotic agents during entombment. An object exhibiting unusually severe deterioration (fig. 7a–b) showed cell wall characteristics that did not resemble any known forms of deterioration. Cell wall layers were not distinct and appeared as disrupted amorphous strands (fig. 7a–b). Some cavities were present in the altered wall material. Although no records of treatments made to the wood during excavation in 1913 exist, it is apparent that the wood had been modified. Many different treatments utilized during the late 19th to early 20th century for consolidation could be responsible for the unusual condition (Hatchfield and Koestler 1987; Mace and Winlock 1916). Most likely, the wood statuette was decayed to a considerable extent by soft rot fungi, as evidenced by the cavities within the cell walls. However, the severely altered state of the wood cells suggests that subsequent treatments accentuated the defect and resulted in what appears to be an unusual form of deterioration. A proper understanding of wood degradation and the devastating effects that some treatment can have on deteriorated wood should help to avoid this problematic situation.
|