ASPECTS OF CHEMICAL RESEARCH IN CONSERVATION: THE DETERIORATION PROCESS
ROBERT L. FELLER
ABSTRACT—Before safe and effective practices in conservation care and treatment can be introduced, the chemist is called upon to address numerous aspects of the proposed innovations. The effort to provide the necessary background of facts and understanding regarding the underlying chemistry may not be of immediate interest to the practicing conservator, and the objective of many such studies is rarely that of immediate application. Examples are cited of research intended to address several different aspects of conservation chemistry. Investigations designed specifically toward the practical evolution of new methods and materials are commonly designated as developmental research, an area that in the past has received too little attention.Accelerated-aging studies indicate that the properties of systems of materials, principally those composed of organic substances, tend to follow distinctive patterns of deterioration—an accelerating, constant, or retarding rate over time or perhaps one that exhibits a period of relatively little change (an induction time) before decided changes occur. It has not yet become a regular custom to describe or approach the deterioration of specific artifactual materials in such terms. One reason may be that deterioration under normal conditions of aging can require a decade or more before marked changes in properties are apparent, so that the pattern of decay over time is not readily recognized. Nevertheless, progress is being made in identifying specific instances of autocatalytic (accelerating) behavior and in monitoring evidences of chemical changes during an apparent induction time. Recent research regarding cellulose triacetate movie film and artifacts of cellulose nitrate provides appropriate examples.
1 INTRODUCTION
Conservators often complain that many papers presented by scientists do not appear to have much relevance to their work. This is undoubtedly the case. The truth of the matter is that our science-based understanding of how to treat and how best to take care of the diverse materials found in museums, archives, and historic sites is still in a very incomplete state. There is a great need to build up the extensive background of facts and understanding required before sound new practices of care and treatment can be established. Considering that the conservation of historic and artistic works encompasses three explicit functions—examination, preservation, and restoration—it is fair to say the contributions of science thus far have been greatest in the area of examination, brought about through the application of an ever-growing list of advanced techniques of analysis. In contrast, our knowledge and understanding of how complex systems of materials deteriorate and, based on this foundation, how they may be treated most effectively contain a multitude of unanswered questions.
2 VARIOUS RESEARCH OBJECTIVES
Some papers that one may encounter in journals and symposia represent an effort to transfer into conservation practice techniques already well-developed in other fields. In the presentations that follow, Walter McCrone's (1994) discussion of the use of polarized light microscopy or Chandra Reedy's (1994) discussion of thin-section petrography provide examples. Other contributions suggest the potential utility of new methods and techniques: Michele Derrick et al. (1994) discuss several possibilities, and Marion Mecklenburg et al. (1994) present the potential insights offered by computerized modeling of stress development. Still others seek to establish a foundation upon which solid advances may be made; Hansen's consideration of the effect of solvents on the physical properties of thermoplastic coatings (Hansen et al. 1991) and Michalski's (1990) proposed model of cleaning (varnish removal by solvent action) are of this type. Other contributions seek to establish general principles that can guide our thinking about a variety of materials and situations: the concept of solubility parameter (regarding solvent action) and Pourbaix diagrams (regarding the corrosion of metals) are prime examples. In any number of fields, a time-honored service has been to identify and begin to fill in significant gaps in current knowledge (Howie 1992; Williams 1993; Wilhelm 1993). A major area of activity aims principally to refine and enlarge upon presently available information; such would be the determination of both number average and weight average molecular weights of familiar thermoplastic resins, more precise data than had been available hitherto (de la Rie 1987). Table 1 summarizes just a few of the numerous objectives behind the research contributions that one will encounter.
TABLE 1 SOME RESEARCH OBJECTIVES IN CONSERVATION SCIENCE
The conservator probably does not see much in this list likely to be of immediate assistance in his or her work, partly because a significant investigative activity is missing: development. Calls for fulfillment of national needs in research and development (R and D) are familiar. Developmental research concerns the myriad problems associated with making untried ideas a practical success. Developmental research involves mission-oriented research, “the practical application of basic knowledge to attaining a specific goal” (NCAC 1979). The objectives cited in table 1 are not those immediately oriented toward the development of practical applications. They represent instead various aspects of the effort to build up the background of information needed to develop safe, sound, innovative, and practical methods of conservation.
A decade ago the effort devoted to developmental research in conservation was limited. Perhaps long-standing policies and practices of funding made it more difficult to obtain grants for development than for the acquisition of basic facts, information, and understanding. In addition, there were far fewer scientists in the conservation field than there are today.
Things have definitely changed. One finds increasing numbers of laboratories dedicated to support for developmental research in specific areas of conservation. Among others, there are now an Image Permanence Institute, a Leather Conservation Centre, a Laboratoire de Recherches des Monuments Historiques, Centro di Studio Cause di Deperimento e Metodi di Conservazione della Opere d'Arte, and a Centre for Archival Polymeric Materials. Most notable, of course, has been the major commitment of the Library of Congress and others toward developing a method of mass deacidification (Luner 1990). There is also considerable interest internationally regarding practical solutions to the consolidation of building stone (Baer et al. 1991; Rodrigues et al. 1992). The Getty Conservation Institute is currently interested in ways of preserving adobe.
Among these efforts are many basic studies undertaken to obtain supporting information. Does deacidification with calcium hydroxide protect paper against mold growth (Marconi 1989)? Does quartz or calcite interfere with the consolidation of stone by methyltrimethoxysilane (Denehey et al. 1992)? Such inquiries are rarely intended or ready for immediate application by the practicing conservator. Let us also admit that scientists often do not make their objectives clear. Nor is it always a simple matter to explain how a contribution is intended to fit into the larger picture. Perhaps it is assumed that these points usually should be rather obvious. It might be a useful exercise, nonetheless, upon being confronted with a rather involved report by a scientist, to speculate as to just what category of topic, such as those in table 1, it is intended to address. There is a further basic problem facing authors in this field: their presentation often must satisfy an extremely wide range of interested parties, extending from museum directors, curators, and practicing conservators to a peer group of conservation scientists as well as specialists in industry and academia. It is virtually impossible to accommodate all these interests in a single presentation.
There are not many occasions in which the research findings proceed directly to the practical end. Caution is certainly commendable if not mandatory. The case of soluble nylon provides a striking example in which a material was introduced directly into conservation practice. Sease (1981) and Hanna and Lee (1988) note that soluble nylon was first mentioned in 1958, recommended largely on the basis of some of its initial properties. Curiously, extensive aging tests were never carried out, nor was background information provided concerning whether this particular chemical substance was likely to be stable. It was more than a decade before a formal publication drew attention to potential problems (DeWitte 1975).
3 A GAP IN PRESENT KNOWLEDGE? PATTERNS OF DETERIORATION
Conservators are fundamentally concerned with retarding the inevitable deterioration of materials. Yet, in this year of 1994, just what is it that we are able to say in answer to the basic question: How do the physical properties of specific organic substances, or systems of such materials, decline with age? If there is not a serious gap, certainly our knowledge of the subject remains limited. There is a general sense that deterioration is rarely a linear process, i.e., that the readily observed changes in properties seldom proceed at a steady rate over time. One may cite many examples, such as those in figure 1, in which plastics and protective coatings initially increase in strength before their properties begin to decline, a decidedly nonlinear pattern of aging (Williams 1993). (This behavior is not universal but is understandable when it occurs. The initial increase in the strength of coatings can come about through cross-linking or the fact that volatile components are lost.) Perhaps Shakespeare's Seven Ages of Man can serve to remind us that it is seldom “all downhill from day one”: the child first grows toward the prime of life; only thereafter does decline set in.
From what is presently known regarding the phenomenon of deterioration, it seems possible to make two statements of general principle: during aging, changes in properties usually (1) are nonlinear over time, and (2) tend to occur in stages. Figure 2, published some years ago (Kelleher 1966; Feller 1977), proposes four basic ways in which the chemical processes of deterioration in organic materials may take place over time. In some cases, perhaps infrequently, the change really does occur in a linear manner (fig. 2a). Far more commonly one observes processes speeding up in time (fig. 2b) or apparently slowing down (fig. 2c). The apparent slowing down of fading or loss of molecular weight is often simply the case of a constant fraction of colorant being lost or chemical bonds being broken in a given unit of time—“first order” decay (Johnston-Feller 1986). Other aging behavior may exhibit a combination of these characteristics (fig. 2d). Kelleher (1966) referred to the manner in which oxygen might be consumed in organic materials. Nevertheless as in chemical processes, changes in physical properties—yellowness and embrittlement—often follow similar patterns.
It is one of the present limitations in our knowledge that we are seldom in a position to state with confidence that the fading of a given dye-substrate system or the embrittlement of a particular type of textile or paper will follow one or the other of these patterns. Perhaps, with a little consideration, the field can make a few such statements, but it has not been a regular custom to look at deterioration problems from the point of view of figure 2. Williams (1993) has urged that increased attention be given to the subject.
A basic factor in our not yet being able to assign particular examples of deterioration to one of these patterns is the matter of the time scale over which deterioration takes place under the mild conditions encountered in museums and archives. In the laboratory, one can usually characterize a pattern of behavior because the full course of deterioration often can be observed, from start to finish, in a matter of hours or weeks. But under museum situations, detecting and demonstrating a change in properties may require monitoring the condition of an artifact over many decades. Naum Gabo's and Antoine Pevsner's sculptures in cellulose nitrate, done in the 1920s, only began to show clear signs of deterioration after some 25 to 60 years had passed (Derrick et al. 1993). Initial studies regarding the lamination of documents in cellulose acetate were made over the period from 1937 to 1959; it was another 16 years (about 1976), however, before observations of poor performance led the Library of Congress to recommend that the practice be discontinued (Stiber 1988). Cellulose triacetate–based motion picture film reportedly showed signs of serious deterioration some 30 to 40 years after its introduction (Edge et. al 1992). On such a time scale, it is not surprising that patterns of deterioration in terms of figure 2 are not broadly recognized.
4 BASIC UNDERSTANDING OF PATTERNS OF DETERIORATION
The effort to establish general principles regarding the various ways in which the properties of materials can change over time has great significance to the practicing conservator. First of all, if one wishes to estimate the possible condition of an artifact some time in the future, it is important to understand whether the changes that have already taken place are likely to continue at a steady pace, apparently slow down, or seriously speed up in time (the latter is often labeled autocatalytic or autoaccelerating behavior). The degradation of cellulose triacetate movie film appears to be of the latter character (Allen et al. 1987); so does the deterioration of cellulose nitrate plastics (Shashoua et al. 1992; Derrick et al. 1993). Within a day of opening a vitrine in which cellulose nitrate materials have been kept in reasonably satisfactory condition for a number of years, serious fracturing can occur; the rapid rate of disintegration then becomes a matter requiring immediate attention (Derrick et al. 1993). The autocatalytic stage can be said to have begun with the opening of the vitrine. Research must attempt to answer many questions: Why do the physical changes occur? Can the behavior be successfully retarded? Can it be circumvented? Is it too late to carry out satisfactory treatment once a particular stage of deterioration has been reached?
The practical importance to the conservator in realizing that a particular deterioration process tends to speed up in time is to emphasize noticing the process and initiating treatment at the earliest possible stage, catching potential trouble at an early point in time. Moreover, when devising accelerated-aging tests to evaluate the potential stability of different types of materials, one must appreciate that it is fundamentally a difficult task to evaluate the relative merit of those that age in the manner of figure 2b versus 2c or 2d.
5 INDUCTION-TIME BEHAVIOR
Of considerable interest is the phenomenon of induction time (fig. 2d): a “time during which no observable change occurs in a chemical reaction or in a physical property, subsequently followed by such a change” (Alger 1989, 46). If conservators observe the condition of an artifact during an induction period and this period is a feature of its particular aging behavior, they may be lulled into thinking that changes are not occurring and that the artifact is in no imminent danger of change, only to find, a relatively short time thereafter, that decided changes have become apparent. Thus, as an initial reaction to a frustrating problem, Calmes (1993, 98) has been led to say of cellulose nitrate: “There appears to be no intermediate stage between the time when the film is in good condition and the time when it is obviously deteriorated.” We now know that research has been able to detect a number of intermediate stages.
One of the earliest examples of induction time that the author encountered is in the cross-linking of butyl methacrylate polymers. When the data reported many years ago are considered (fig. 3), one sees that there is an apparent induction period of 11 years before insoluble (cross-linked) matter was formed in the various butyl methacrylate–based coatings that had been exposed under skylights on the wall of the laboratory of the Fogg Art Museum (Feller 1976; Feller et al. 1985). If these coatings had been tested for insoluble matter after eight years, the conservator might have concluded that they had no tendency to cross-link. Yet, a decade later, there would have been convincing evidence that insoluble matter had begun to form. Basic scientific studies and principles support these observations; the theory of how cross-linking takes place leads one to expect the existence of an induction period.
Fig. 3.
Insoluble matter found in n-butyl and isoamyl copolymers exposed on a well-illuminated wall of the Fogg Art Museum laboratory (Feller 1976)
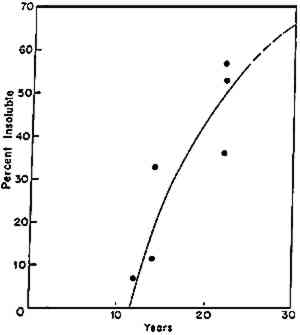 |
The chalking of paints—i.e., the degradation of the vehicle so that the paint begins to flake from the surface—has also been observed to exhibit induction-time behavior (Feller 1974). A situation that resembles this behavior is also encountered in the corrosion of metals that have been protected by a paint or varnish. Graphs of arbitrary “corrosion ratings” often show a period of time in which no noticeable corrosion has occurred. The explanation seems plain enough: corrosion of the metal substrate tends not to occur until the organic coating has begun to fail. This situation need not fit a rigorous definition of induction-time chemistry. Yet from the practical point of view of persons responsible for the care of metal artifacts, there is a time when all goes well. Thereafter, a moment occurs at some point in time, often difficult to predict or to “catch,” at which corrosion becomes evident.
When these concepts were initially being reviewed in 1972–77, it was possible to suggest that “most of the measures that are taken, or that should be taken, to conserve organic material are concerned with processes that occur during the induction time of oxidative deterioration and are measures essentially concerned with prolongation of the period of induction” (Feller 1974, 146). This point of view is well understood by polymer chemists. Most recently, Edge et al. (1992, 148) stated again, “It is obvious that the small physico-chemical changes which occur during the early stages of aging, i.e., during the induction period prior to the onset of degradation, will be of prime importance with respect to subsequent stability.” Although specific instances can be documented, the phenomenon of apparent induction time is not universally encountered in deterioration problems. When it does occur, however, it is of fundamental significance in preventive care.
6 EARLY DETECTION OF IMPENDING DETERIORATION
It is possible that the subtle processes taking place during the “apparent induction period” can be monitored successfully. In the case of rubber cement, it was shown that an increase in weight and in hydroperoxide concentration could be detected before serious discoloration took place (Feller and Enke 1987). Moreover, a “sticky” stage was also observed before serious loss of solubility took place. These observations held out the possibility that one could find and measure properties that were changing during a period when few or no visually observable changes were occurring. More recently, Shashoua et al. (1992) report that they are able to follow the early loss of nitro (NO2) groups relative to carbonyl (CO) in the infrared spectra of nitrocellulose adhesives that had been applied to objects over a period of 30 years. The loss of the camphor plasticizer can be similarly monitored (Derrick et al. 1993). There are also early stages in which odors are detectable or exudations observed. Perhaps one day these emissions can be precisely measured to monitor condition.
Studies regarding the deterioration of cellulose triacetate motion picture film, representing more than 30 man-years of effort at the Centre for Archival Polymeric Materials, Manchester, England, have confirmed that the process is autocatalytic and that it tends to exhibit an induction period. The Centre has since demonstrated that early changes within the film can be monitored by analysis of the moisture content by means of a “novel technique of microwave spectroscopy,” a nondestructive method (Edge et al. 1992, 147). This example and that of the cellulose nitrate studies are cited to indicate that progress is indeed currently being made in our ability to monitor the subtle changes that can take place in organic materials before serious physical deterioration sets in.
7 CONCLUSIONS
Many research contributions are not intended for immediate application by the practicing conservator. Before sound practices of prevention and treatment can be developed, extensive background information needs to be acquired regarding the way that materials and systems of materials can be characterized and how and why specific materials behave as they do. Only then can research be directed effectively toward the development of practical techniques and procedures.
Deficiencies remain in our understanding of the task of retarding deterioration, and they are the concern of all entrusted with the care of collections. One particular aspect concerns the pattern of degradation that specific classes of materials undergo. One might call this an understanding of the “pathogenesis” of degradation, or as Williams (1993, 140) calls it, “the degradation profile,” as illustrated in figure 2. Increasingly, conservation scientists are beginning to consider deterioration processes from this point of view. Thus, in spite of the long time scale over which the deterioration of museum artifacts usually takes place, the fact that certain specific materials degrade autocatalytically is now well recognized. Moreover, in situations in which an induction period seems to be part of the picture, techniques are being developed to monitor the chemical changes that occur during this deceptively quiescent period.
REFERENCES
Alger, M.S.M.1989. In Polymer Science Dictionary. London and New York: Elsevier Applied Science.
Allen, N. S., M.Edge, J. H.Appleyard, T. S.Jewitt, C. V.Horie, and D.Francis. 1987. Degradation of historic cellulose triacetate cinematographic film: The vinegar syndrome. Polymer Degradation and Stability19:379–87.
Baer, N. S., C.Sabbioni, and A. I.Sors, eds. 1991. Science technology and the European cultural heritage: Proceedings of the European symposium, Bologna, Italy, 13–16 June 1989. Guildford, England: Butterworth-Heinemann.
Calmes, A.1993. Plastics found in archives. In Saving the twentieth century: The conservation of modern materials, ed. D.Grattan. Ottawa: Canadian Conservation Institute. 95–102.
de laRie, R.1987. The influence of varnishes on the appearence of paintings. Studies in Conservation32:1–13.
Denehey, C., G. S. Wheeler, and S.-C.Su. 1992. The influence of quartz and calcite on the polymerization of methyltrimethoxysilane. In Proceedings of the 7th international congress on deterioration and conservation of stone, ed. J. D.Rodrigues, F.Henriques, F. J.Jermias, and F.Telmo. Held in Lisbon, Portugal, June 15–18, 1992. 1043–1052.
Derrick, M. R., E. F.Doehne, A. E.Parker, and D. C.Stulik. 1994. Some new analytical techniques for use in conservation. Journal of the American Institute for Conservation33:171–84.
Derrick, M., D.Stulik, and E.Ordonez. 1993. Deterioration of cellulose nitrate sculptures made by Gabo and Pevsner. In Saving the twentieth century: The conservation of modern materials, ed. D.Grattan. Ottawa: Canadian Conservation Institute. 169–82.
DeWitte, E.1975. Soluble nylon as consolidation agent for stone. Studies in Conservation20:30–34.
Edge, M., N. S.Allen, D. A. R.Williams, F.Thomson, and V.Horie. 1992. Methods of predicting stability testing of archival polymers: A preliminary assessment of cellulose triacetate based motion picture film. Polymer degradation and stability35:147–55.
Feller, R. L.1974. Fundamentals of conservation science: Induction time and the auto-oxidation of organic compounds. Bulletin of the American Institute for Conservation14(2):142–51.
Feller, R. L.1976. The deterioration of organic substance and the analysis of paints and varnishes. In Preservation and conservation: Principles and practices,ed. S.Timmons. Washington, D.C.: Smithsonian Institution Press. 287–98.
Feller, R. L.1977. Stages in the deterioration of organic materials. In Preservation of paper and textiles of historic and artistic value, ed. J. C. Williams. Advances in Chemistry series 164. Washington, D.C.: American Chemical Society. 314–35.
Feller, R. L., and D. B.Enke. 1982. Stages in deterioration: The examples of rubber cement and transparent tape. In Science and technology in the service of conservation, ed. N. S. Brommelle and G. Thomson.London: International Institute for Conservation of Historic and Artistic Works. 19–23.
Feller, R. L., N.Stolow, and E. H. Jones. 1985. On picture varnishes and their solvents, rev. and enlarged ed. Washington, D.C.: National Gallery of Art. 158–59.
Hanna, S. B., and N. J. Lee. 1988. The consequences of previous adhesives and consolidants used for stone conservation at the British Museum. In Early advances in conservation, ed. V.Daniels. British Museum Occasional Paper 65. 89–102.
Hansen, E. F., M. R.Derrick, M. R.Schilling, and R.Garcia. 1991. The effects of solution application on some mechanical and physical properties of thermoplastic amorphous polymers used in conservation: Poly(vinyl acetate)s. Journal of the American Institute for Conservation30:203–13.
Howie, F. M., ed. 1992. The care and conservation of geological material: Minerals, rocks, meteorites and lunar finds. Guildford, England: Butterworth-Heinemann.
Jain, R. K., B. K.Saxena, G. D.Bansal, and K. K.Asthana. 1983. Weathering behaviour of glass fibre reinforced polester sheets. Durability of building materials2:27–36.
Johnston-Feller, R. M.1986. Reflections on the phenomenon of fading. Journal of Coatings Technology58 (736):32–50.
Kelleher, P. G.1966. Thermal oxidation of thermoplastics. Journal of Applied Polymer Science10: 843–57.
Luner, P., ed. 1990. Paper preservation: Current issues and recent developments. Atlanta: Tappi Press.
Marconi, C.1989. Influenza della deacidificazione acquosa con idrossido di calcio nei riguardi della resistenza della carte Whatman all attacco di miceta carticoli. Bollettino dell Istituto Centrale per la Patologia del Libro Alfonso Gallo43:177–84.
McCrone, W. C.1994. The use of polarized light microscopy in conservation. Journal of the American Institute for Conservation33:101–14.
Mecklenberg, M. F., M.McCormick-Goodhart, and C. S.Tumosa. 1994. Investigation into the deterioration of paintings and photographs using computerized modeling of stress development. Journal of the American Institute for Conservation33:153–70.
Michalski, S.1990. A physical model of the cleaning of oil paint. In Cleaning, retouching, and coatings, ed. J. S.Mills, and P.Smith. London: International Institute for Conservation of Historic and Artistic Works. 85–92.
NCAC. 1979. Report of the study committee on scientific support for conservation of cultural property, Washington, D.C.: National Conservation Advisory Council.
Reedy, C. L.1994. Thin-section petrography in studies of cultural materials. Journal of the American Institute for Conservation. 33:115–29.
Rodrigues, J. D., F.Henriques, and F. T.Jermias, eds. 1992. Proceedings of the 7th international congress on deterioration and conservation of stone,. Held in Lisbon, Portugal, June 15–18, 1992. 1043–1052.
Sease, C.1981. The case against using soluble nylon in conservation work. Studies in Conservation26:102–10.
Shashoua, Y., S. M.Bradley, and V. D.Daniels. 1992. Degradation of cellulose nitrate adhesive. Studies in Conservation87:113–19.
Stiber, L. 1988. The delamination of the Washington and Lee ledger, part 1. An overview of cellulose acetate lamination. In Early advances in conservation, ed. V.Daniels. British Museum Occasional Paper65. 27–40.
Wilhelm, H. 1993. The permanence and care of color photographs. Grinnell, Ia.:Preservation Publishing Co.
Williams, R. S.1993. Composition implications of plastic artifacts: A survey of additives and their effects on longevity of plastics. In Saving the twentieth century: The conservation of modern materials, ed. D.Grattan. Ottawa: Canadian Conservation Institute. 135–53.
Winslow, F. H., and W. L.Hawkins. 1967. Some weathering characteristics of plastics. In Weatherability of plastic materials, ed. M. R. Kamal. Applied Polymer Symposia 4. New York: J. Wiley. 29–39.
AUTHOR INFORMATION
ROBERT L. FELLER received a Ph.D. in physical-organic chemistry in 1950 and became engaged in research on conservation-related problems immediately thereafter, initially as senior fellow of the National Gallery of Art Research Project and later as director of the Research Center on the Materials of the Artist and Conservator at Mellon Institute (now Carnegie Mellon Research Institute), Pittsburgh. His principal research interests have concerned the physical properties and long-term stability of thermoplastic resins and the fading of pigments and other deteriorating action of visible and ultraviolet radiation. An Honorary Member of the American Institute for Conservation, he is director emeritus of the Research Center following retirement in 1988. Address: Carnegie Mellon Research Institute, 4400 Fifth Ave., Pittsburgh, Pa. 15213.
Section Index |