GAS CHROMATOGRAPHIC ANALYSIS OF AMINO ACIDS AS ETHYL CHLOROFORMATE DERIVATIVES.
MICHAEL R. SCHILLING, HERANT P. KHANJIAN, & LUIZ A. C. SOUZA
ABSTRACT—Gas chromatography has long been used as a means of identifying proteins in acid hydrolysates of paints and adhesives on the basis of their amino acid composition. Existing procedures for amino acid derivatization can, however, take up to 4 hours per analysis, whereas a new procedure employed at the Getty Conservation Institute can be carried out in less than 20 minutes. This procedure involves the formation of N(O,S)-ethoxycarbonyl ethyl esters of amino acids using ethyl chloroformate and then a separation on an HP-INNOWAX capillary column. This procedure was employed to analyze samples from the binding media library at the Getty Conservation Institute, and amino acid compositions were tabulated for various proteins that could be used for identification purposes.
1 INTRODUCTION
Proteins are an important class of natural organic materials that have long been used as binding media and adhesives. Of the many proteins found in nature, the ones encountered most frequently in conservation include those of glue (obtained by boiling animal hides and bones), size (a more purified form of glue), casein (present in milk), glair (egg white), and egg yolk (K�hn 1986).
In essence, proteins are biopolymers of amino acids. Amino acids have the general formula H2N-CHR-CO2H, where R is a functional group that imparts unique chemical and physical properties. Proteins may be broken down into their constituent amino acids by the action of strong aqueous acid. Either liquid acid or acid vapor may be used for this purpose (Pickering and Newton 1990). This process, known as hydrolysis, is an important first step in many of the analytical methods used for identification of proteins. Twenty natural amino acids are commonly found in protein hydrolysates, and many others have been identified (see appendix 1).
One way that proteins differ from each other is in their relative proportions of amino acids. For example, glue is characterized by high levels of hydroxyproline, glycine, and alanine, while casein contains large amounts of aspartic acid and glutamic acid but no hydroxyproline. Amino acid composition is often used as the basis for identification of proteins.
A number of analytical methods can be used to identify proteins directly, or through their acid hydrolysates, including spot tests (Collings and Young 1976; Stulik and Florsheim 1992); thin-layer chromatography (Masschelein-Kleiner 1974; Striegel 1994); high-performance liquid chromatography (HPLC) (Sinkai and Sugisita 1990; Halpine 1992; Grzywacz 1994; Ronca 1994); and ion chromatography (Keck and Peters 1969; Sack et al. 1981; Pickering 1989).
Gas chromatography (GC) is an analytical technique that has been applied to study all the major classes of organic binding media. Overviews of the general procedures may be found in Mills and White (1978, 1987) and in Pancella and Bart (1989). These methods have greatly enhanced our existing knowledge of the nature of binding media and their uses by artists.
A number of GC procedures have been developed for identification of proteins. Before GC analysis, amino acids in protein hydrolysates must first be converted to volatile derivatives; this conversion requires reaction of both the amine and the carboxylic acid functional groups. Various authors have taken different approaches to derivatization, yielding results that have not been completely satisfactory (Masschelein-Kleiner 1976; White 1984; Pancella and Bart 1989).
The main difficulty with all existing GC methods reported in the conservation literature is excessive sample preparation time. Derivatization may require from 4 to 24 hours, involving many tedious chemical reactions with the potential for analyte losses at each transfer step. Clearly, a need exists for a rapid derivatization method that is suitable for analysis of protein present in art objects and artifacts. In a procedure for analyzing acid hydrolysates of blood serum specimens, Hušek (1991) used ethyl chloroformate (ECF) to form N(O,S)-ethoxycarbonyl amino acid ethyl esters via the following reaction:
Fig. .
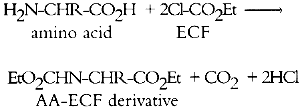 |
Utilizing split injection of a chloroform solution of the derivatives onto a capillary GC column coated with OV-1701 cyanopropyl stationary phase for separation, total derivatization and analysis time was 4 minutes. Nineteen of the common amino acids were quantified by this method, with the exception of arginine, which irreversibly adsorbs onto the column because the imino group on the guanidine moiety fails to derivatize. Nonetheless, the technique (hereafter referred to as the ECF method) satisfied the criterion of minimal sample preparation and analysis time and warranted investigation for possible uses in conservation.
Because proteins are identified by comparing amino acid compositions of unknown samples to known materials, it is important to have access to a source of amino acid data for commonly encountered proteinaceous substances. Proteins associated with art objects, artifacts, and monuments may come from various sources. As mentioned earlier, binding media and adhesives are major uses of proteinaceous materials. Besides glue, casein, and egg, other materials have traditionally been used as binding media and adhesives that may contain small amounts of amino acids or proteins, such as wheat starch, rice starch, and blood (Sinkai and Sugisita 1990). Other organic media that are not normally considered proteinaceous may contain small but measurable quantities of amino acids. For instance, certain plant gums are composed of glycoproteins (Sharon 1975).
Samples from objects may contain amino acids that originate from ubiquitous proteinaceous contaminants. Handling of objects leads to transfer of small but measurable quantities of amino acids present in skin, such as alanine, valine, leucine, glycine, proline, aspartic acid, threonine, serine, lysine, and histidine (Hamilton 1965). Outdoor objects and historic buildings may be exposed to microbes (Ronca 1994), guano, and other proteinaceous environmental contaminants. The cleaning action of saliva on painted objects is directly attributable to the presence of enzymes; after treatment, the enzymes may remain on the painted surface (Rom�o et al. 1990). Over time the contamination of objects with proteinaceous substances is a virtual certainty, resulting in confusing amino acid compositions.
In general, it is difficult to locate amino acid concentration data on the wide variety of proteins that are associated with the conservation of works of art. Even the excellent compilation by Mills and White (1987) lacks information on proteinaceous contaminants. Thus, an important aspect of this paper is a tabulation of amino acid compositional data for proteins that a conservation scientist may routinely encounter.
2 SCOPE OF RESEARCH
The split injection technique employed by Hušek (1991) could not be used in the analysis of paint samples from objects. Excessively high detection limits result from split injection because only a small fraction of the sample solution is transferred to the GC column (the remainder is diverted to a vent).
Much lower detection limits may be achieved using splitless injection, a technique in which nearly the entire sample is deposited into the GC column for analysis. To use splitless injection, it is necessary to reduce the initial temperature of the oven to a value below the boiling point of the solvent, modify the GC temperature program, increase the helium flow rate through the column, and use a column that is compatible with the solvent. Trial usage in our laboratory of an untested splitless injection procedure (Hušek 1991) resulted in premature failure of the OV-1701 capillary GC column, caused by stripping of the stationary phase by the chloroform and acid components of the derivatization solvent. Eventually, a satisfactory procedure was developed that exhibited the requisite sensitivity for analysis of samples from art objects without causing unnecessary damage to the capillary GC column.
The amino acid composition data appearing in this article were obtained from various sources. From a thorough study of the conservation literature, a number of references were located that contained the amino acid compositions for common proteinaceous binding media and adhesives. The data in these articles were obtained by various analytical techniques: ion chromatography (Keck and Peters 1969), high-performance liquid chromatography (Sinkai and Sugisita 1990), and elemental analysis (Bergquist 1981; Hall 1981).
To augment the existing literature data, the ECF method was used to analyze a large number of proteinaceous materials. These samples were collected from various sources for testing; the primary source was the binding media library of the Getty Conservation Institute (Stulik et al. 1991). This library consists of more than 1,550 samples of modern and historical organic binding media donated by individuals and institutions or obtained from vendors; it includes 320 proteinaceous materials. The collection exists for the purpose of advanced scientific study and is especially useful as a source of materials for research.
3 RESULTS
Complete procedures for vapor-phase hydrolysis of proteins, derivatization of amino acids using ECF, and GC analysis with splitless injection and flame ionization detection (FID) are outlined in appendix 2. Information required to keep the procedure working reliably on a daily basis is described in appendix 3. Figure 1, an overlay of the analytical results for the amino acid calibration standard and a blank, shows that all the ECF-derivatized amino acids are resolved well. Although detection of histidine, tyrosine, and cysteine was possible, peaks for these amino acids do not exhibit the reproducibility required for proper quantitation. As stated earlier, because arginine does not derivatize by ECF, it cannot be detected. Figure 2 shows the chromatograms resulting from the analysis of whole egg, rabbit skin glue, and casein.
Fig. 1.
Capillary gas chromatograms (scaling arbitrary) for ECF-derivatives of amino acids present in the Sigma A2161 calibration standard, and the analysis of an empty vial that served as a blank. The peaks for lysine (K), histidine (H), and tyrosine (Y) are indicated for the standard. Peaks for the remaining amino acids are identified in figure 2.
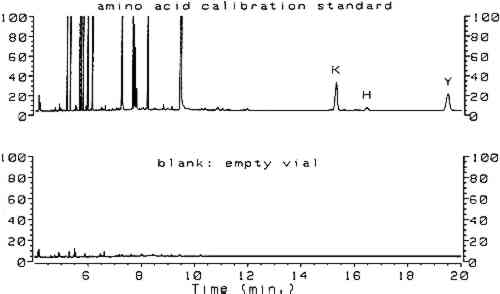 |
Fig. 2.
Capillary gas chromatograms (scaling arbitrary) for ECF-derivatives of amino acids present in hydrolysates of whole egg, rabbit skin glue, and casein. Peaks for amino acids are labeled according to the list of abbreviations in appendix 1. The peak for lysine at 15.3 minutes was not shown to permit better clarity. Peaks for ethyl esters of azelaic acid, palmitic acid, stearic acid, and oleic acid appear in the chromatogram for whole egg at 6.35, 6.5, 7.18, and 7.27 minutes, respectively.
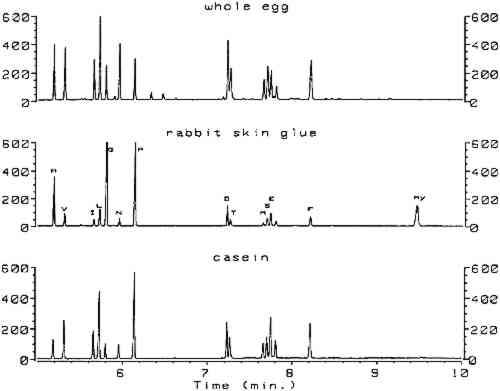 |
All protein amino acid compositions reported in tables 1, 2, and 3, including those obtained from the literature, are expressed in mole percent of only those amino acids detected by the ECF method. In addition, the weight percent of protein present in the samples could be estimated from the sum of the weight percentages of all ECF-derivatized amino acids detected. To correct for losses in the hydrolysis reaction, the weight percent of protein was normalized to the yield obtained for a sample of rabbit skin glue that was included in a separate vial with each set of sample vials. Typical hydrolysis yields for rabbit skin glue were 85–95% of all ECF-derivatized amino acids detected. The recovery of norleucine internal standard was also calculated in order to monitor transfer losses and solvent volume changes.
TABLE 1 AMINO ACID COMPOSITION DATA FROM VARIOUS SOURCES FOR SELECTED PROTEINS
TABLE 2 AMINO ACID COMPOSITION DATA FROM VARIOUS SOURCES FOR SELECTED EGG PROTEINS
TABLE 3 AMINO ACID COMPOSITION DATA FROM VARIOUS SOURCES FOR SELECTED PROTEINS
Table 1 summarizes the amino acid composition data for casein, glue, and gelatin samples. Data for egg proteins are listed in table 2. The results for blood, plant gums, other proteins, and proteinaceous contaminants are listed in table 3. In general, although the data listed in tables 1, 2, and 3 were acquired using several different analytical techniques, surprisingly good agreement was demonstrated. This fact illustrates that all the analytical techniques work well for pure, unpigmented samples of fresh protein.
Interesting results were obtained from the analysis of common plant gums (see table 3). All gums tested contained low levels of amino acids. Furthermore, low norleucine internal standard recoveries were consistent with observations that condensation products can form between the carbohydrates and the free amino acids, reducing overall yields (White 1984). However, the most significant observation was that hydroxyproline was present in every gum tested, in concentrations of up to 29 mole percent for samples of gum arabic.
4 DISCUSSION
The primary change made in the derivatization procedure of Hušek (1991) involved elimination of chloroform, water, and acid from the injection solvent, so that stationary phase stripping of the column would not occur. This procedure involved transferring the chloroform solution of the derivatives to a clean vial, extracting the aqueous acid solution a second time with chloroform, drying the combined chloroform layers over sodium sulfate, evaporating the chloroform to dryness, and using benzene as the injection solvent.
Column activity can result from partial stripping of the stationary phase and result in peak resolution problems and tailed peaks. To improve resolution, Hušek (1991) investigated columns coated with a number of different stationary phases and found that OV-1701 gave the best compromise between resolution and analysis time. We found this column to be prone to activity problems, so a number of polar stationary phases (OV-1701, DB-1701, DB-WAX, and HP-INNOWAX) were tested to determine which was most resistant to activity problems. Although all provided adequate resolution, HP-INNOWAX proved to be the most robust and could be heated to the highest temperature to bake out contaminants.
The HP-INNOWAX stationary phase is chemically very similar to CARBOWAX, a stationary phase used for separation of fatty acid methyl esters derived from oils and lipids. It is indeed fortuitous that the capillary column best suited to separate ECF derivatives of amino acids may also be used in the identification of drying oils and lipids. Conservation science laboratories already equipped with a gas chromatograph for oil identification may perform protein identification without the additional expense of obtaining a second column. Furthermore, the inevitable instrument setup delays due to changing columns are eliminated. Most important, it is possible to forgo the major expense of buying HPLC equipment to perform protein identification.
In general, vapor-phase hydrolysis performed satisfactorily for samples below 100 μg in weight. Weight percentages of protein, after correction for the hydrolysis yield by the rabbit skin glue reference standard, were accurate to within 10% relative. Errors were much larger for samples exceeding 100 μg, especially for egg yolk. Presumably the hydrophobic nature of the lipids in egg yolk prevented complete vapor-phase hydrolysis.
Very little contamination was observed in the blank analyses, due in large part to the fact that in the vapor-phase hydrolysis technique, preconcentration of the hydrolysate in order to accommodate low amino acid concentrations is not required. This feature is important for the study of microsamples removed from objects. Identification of the contaminants was not performed in this study.
Of the 20 common amino acids, a few are sensitive to acid hydrolysis conditions (Pickering and Newton 1990). Asparagine and glutamine are converted to aspartic acid and glutamic acid, respectively. Serine and threonine concentrations are reduced by approximately 10% through oxidation; if deemed necessary, corrections in yield can be made. Tryptophan is destroyed completely by hydrolysis. Methionine is converted to methionine sulfoxide unless first reacted with 2-mercaptoethanol. The yield of cysteine can be improved by conversion to cysteic acid with performic acid.
The concentrations of amino acids in protein hydrolysates can be affected by the presence of pigments and other inorganic compounds (Halpine 1992; Grzywacz 1994). These so-called matrix effects must be evaluated thoroughly for a given analytical method to understand which amino acids are free of them and which are most affected by them, so that a reliable protein identification scheme may be developed. The data in tables 1, 2, and 3 were all obtained in the absence of pigments; hence they are useful for reference purposes.
Furthermore, Karpowicz (1981) has shown that certain amino acids are strongly affected by aging (especially methionine and cysteine). Thus, the combined effects of pigments and aging may alter the normal profile of amino acids in paint samples to such an extent that results obtained from normal identification procedures may be compromised. These effects have been studied in relation to the ECF method and are the subject of another paper (Schilling and Khanjian, in preparation).
Compounds other than amino acids may be derivatized by ethyl chloroformate. For example, peaks from ethyl esters of palmitic, stearic, and oleic acid appear in the chromatograms of egg tempera (see fig. 2 for whole egg) and drying oils. The fatty acids originate from the triglycerides and are formed by acid hydrolysis and ECF-esterification. Azelaic acid, formed during the drying of oils rich in linoleic acid or linolenic acid, appears as a prominent peak in samples that contain drying oils (linseed oil, walnut oil, and poppy oil) and is much smaller in chromatograms for egg yolk and whole egg samples (Mills and White 1987).
Oleic acid may be present in sufficiently high concentration in some egg yolk samples to cause an overlap problem with threonine, resulting in erroneously high threonine concentrations. If an exact determination of threonine is required, we found that residual free fatty acids in sample hydrolysates may be extracted with chloroform prior to derivatization. Concentrations of free amino acids, which are nearly insoluble in chloroform, are not affected by the treatment. Extraction was not performed for the samples in this study, so the data for threonine in egg yolk should be considered approximate.
In general, good agreement is exhibited between the literature data and the data obtained by the ECF method, demonstrating that the level of accuracy is high when any of the methods is used to test pure proteinaceous substances. The small variations displayed in the tables may be a result of genuine compositional differences of the materials tested or of actual methodological systematic errors (such as matrix effects, derivatization yields, or interferences). To identify the reasons behind the small discrepancies would require analytical round-robin testing of a set of standard protein reference materials. This step is hardly justified in view of the overall agreement exhibited by the data.
The compositions of gelatin and fish glues tend to be more variable than those of the collagen samples, especially regarding the concentrations of hydroxyproline, alanine, threonine, phenylalanine, and proline. This variability may be due to improper container labeling or may reflect different sources for the gelatin. A few samples of fish glue and isinglass exhibited reduced proline and hydroxyproline, in keeping with observations made by White (1984).
We originally attributed the presence of amino acids in the plant gums to contamination of the samples, a conclusion that Keck and Peters (1969) also reached. But Sharon (1975) noted that amino acids are normally present in plant gums in the form of glycoproteins. Glycoproteins consist of polypeptide chains to which carbohydrate moieties are attached. The amino acids form bonds to specific carbohydrates (for example, arabinose bonds preferentially to hydroxyproline). It was, therefore, not a coincidence that high levels of hydroxyproline should be detected in gum arabic samples, because arabinose is one of the principal sugars of gum arabic.
This information has a substantial impact on the identification of proteins using amino acid composition. One of the principal indicators that glue or gelatin may be present in a sample is a high level of hydroxyproline. Lower levels of hydroxyproline in paint samples are often assumed to originate from particles of glue-based ground inadvertently sampled with the paint. In light of the above results, care should be taken when interpreting amino acid composition data. One way to avoid difficulties is to consider the relative amounts of all amino acids in the sample. As mentioned above, glue contains very high concentrations of glycine and proline in addition to hydroxyproline, while gum arabic does not.
Considering the results from testing of proteinaceous contaminants, and given the likelihood that an object may someday become the subject of an analytical investigation, a few simple precautions should be taken to minimize the accumulation of unwanted proteins. Deposition of amino acids from skin can be reduced by wearing gloves when handling objects (already a common routine). The practice of cleaning painted objects with saliva should be discouraged. Furthermore, the wetting of paintbrushes with saliva to pick up samples for testing must be completely avoided. Unfortunately, little can be done to prevent exposure of outdoor objects to proteinaceous contamination.
An important component of any scheme for identifying proteins is a collection of data for reference materials (such as the composition of eggs or gums). These data may be obtained by in-house testing of proteinaceous materials (if a suitably large collection of proteinaceous materials is available) or by consulting the literature. Only the literature data expressed in terms of weight (or molar) percentages are useful; peak areas or peak area ratios are practically useless outside the laboratory in which they were compiled. Thus, if one is engaged in amino acid analysis of samples removed from art objects, it would be of great service to the conservation community to report amino acid data in terms of concentration. In so doing, conservators can build a significant database that will deepen our understanding of artists and their methods.
5 CONCLUSIONS
The results demonstrate that protein present in art objects or used in conservation may be identified on the basis of their amino acid composition through gas chromatographic analysis of ethyl chloroformate derivatives. The method is rapid, is sensitive to low concentrations of amino acids, and affords data that are consistent with HPLC and ion chromatography results reported in the literature. Detection of significant amounts of hydroxyproline in many plant gums, especially gum arabic, complicates the identification of glue and gelatin in paint and adhesives. The relative amounts of all amino acids should be taken into consideration in order to avoid misinterpretation of results.
ACKNOWLEDGEMENTS
The authors wish to thank the following members of the Scientific Program of the Getty Conservation Institute (GCI): Dusan Stulik, head, Analytical Section, who developed the binding medium project and supported this research; and James Druzik, William Ginell, David Scott, and Charles Selwitz, who kindly reviewed this manuscript. Special thanks go to Art Mendicino, gas chromatography specialist at Ohio Valley Specialty Chemical, Inc., who provided invaluable information on column stability and graciously supplied a number of replacement GC columns. Thanks also go to the National Research Council of Brazil for supporting Luiz Souza's research fellowship at the GCI; to Adriano Ramos, with whom Souza had valuable discussions about the materials and methods of polychromed sculpture manufacture; and to Michele Derrick, former GCI associate scientist, who offered advice and encouragement.
APPENDIX
1 APPENDIX 1: AMINO ACIDS THAT MAY BE FOUND IN ACID HYDROLYSATES OF PROTEINS
2 APPENDIX 2: EXPERIMENTAL PROCEDURE
2.1 VAPOR-PHASE ACID HYDROLYSIS
As a precaution, gloves should be worn during all sample handling steps. Samples were weighed onto small, V-shaped aluminum pans to the nearest 0.1 μg using a microbalance. Prior to analysis, the samples of saliva, guano, and blood were dried at room temperature for 48 hours. Samples ranged from 50 to 200 μg. After being weighed, samples were transferred to 1 ml glass sample tubes by gentle tapping. Sample tubes (a maximum of 10) were placed inside a 25 ml glass hydrolysis chamber. Accompanying the sample tubes were an empty tube that served as a blank, a tube containing rabbit skin glue that served to monitor the yield of the hydrolysis reaction, and a tube containing 10 μl of a calibration standard. To each tube was added 2 μl of L-norleucine internal standard solution (1,500 ppm in 0.1M HCl).
A 200 μl aliquot of 6M HCl was introduced to the bottom of the chamber, which was subsequently capped tightly with a Mininert valve. To minimize losses of amino acids during hydrolysis due to oxidation, the concentration of oxygen inside the chamber was reduced by thrice alternating between vacuum for 30 seconds (supplied by a mechanical pump) and nitrogen purge for 30 seconds. Access to the atmosphere inside the chamber was provided by penetrating the septum of the Mininert valve with a 20 gauge needle attached to a vacuum hose and a gas-switching valve. The chamber was evacuated for a fourth and final time prior to hydrolysis, then kept at 105�C in an oven for 24–36 hours to fully hydrolyze the samples (Halpine 1992).
After the chamber was cooled, the Mininert valve was unscrewed slowly to release the vacuum, and the outsides of the tubes were wiped dry. To eliminate the last traces of liquid, the tubes were reinserted into a clean, dry chamber, and vacuum was applied for 30 minutes while the chamber was maintained at 50�C. Remaining traces of acid were removed by washing each tube with 15 μl of water and drying them at 50�C in a gentle stream of nitrogen. The hydrolysate was dissolved in 120 μl of 25mM HCl.
2.2 DERIVATIZATION
An aliquot of the hydrolysate was transferred to a 1.2 ml flat-bottomed vial. It is advantageous to retain a portion of the hydrolysate in the event that reanalysis is required. Experience has shown that 60 μl of hydrolysate gives adequate results for all but submicrogram paint samples. The aliquot must contain less than 100 μg of amino acids (Hušek 1991). The solution was adjusted with 25mM HCl to give a final volume of 60 μl.
After mixing the 60 μl hydrolysate aliquot with 32 μl of ethanol and 8 μl of pyridine, 5 μl of ethyl chloroformate was added, and the vial was shaken gently for 5 seconds until evolution of carbon dioxide subsided. A 100 μl aliquot of 1% ECF in chloroform was added to extract the amino acid derivatives. After shaking, the vial was gently tapped to facilitate separation of the two layers (Hušek 1991).
The chloroform layer (usually, but not always, the lower of the two) was transferred to a flat-bottomed vial containing 100 μl of chloroform and 10–20 mg of anhydrous sodium sulfate (to remove traces of water) and then transferred to a third clean, flat-bottomed vial. The solution of ethanol:pyridine:acid hydrolysate was extracted a second time with 100 μl of chloroform. The chloroform layer was transferred to the vial containing sodium sulfate, and the chloroform solutions were combined into the third vial.
The derivatives were concentrated by evaporating the chloroform to dryness under a slow stream of nitrogen. This step must be performed with great care to minimize loss of volatile derivatives. Evaporation of the chloroform is nearly complete when condensation forms on the vial; at this instant the solvent remaining inside the vial is greatly enriched in pyridine, as evidenced by the odor. Complete evaporation may be facilitated by warming the bottom of the vial between the fingertips. A small volume of benzene is added to the vial to dissolve the derivatives prior to injection (5–10 μl was the typical amount for microsamples from objects, whereas 100 μl may be used for larger paint samples and for pure proteinaceous materials). Approximately 20 minutes were required for derivatization and sample preparation. The benzene solutions of the derivatives are stable for at least 2 days when stored at 4�C.
2.3 GAS CHROMATOGRAPH INSTRUMENTAL PARAMETERS
A Hewlett Packard 5890A gas chromatograph equipped with a flame ionization detector, split/splitless inlet, and Pascal ChemStation was used for this study. Amino acid derivatives were separated on a 15 M � 0.25 mm fused silica capillary column coated with a 0.25 μm film of HP-INNOWAX. Helium was used as carrier gas at a nominal linear velocity of 95 cm/second (measured at 70�C and an inlet pressure of 17 psig); the split vent flow rate was 50 ml/minute and the septum purge flow rate was 0.4 ml/minute. Samples were injected into the inlet held at 240�C, with a purge delay of 30 seconds. The oven, maintained at 70�C for 1 minute, was heated at a rate of 27�C/minute to 250�C and held at that temperature for 9.33 minutes. The detector, set to 240�C, was adjusted to detect peaks with widths greater than 0.01 minute. The total analysis time was 17 minutes.
Amino acid calibration standards were prepared by serial dilution of a standard mixture obtained from Sigma Chemical Co. (no. A2161); nominal concentrations ranged from 100 ppm to 0.1 ppm. L-norleucine was added as an internal standard at a concentration of 150 ppm. Aliquots of the calibration standards (10 μl) were derivatized in the same manner as the samples. The derivatives were dissolved in 100 μl of benzene prior to injection. Calibration curves for all analytes exhibited correlation coefficients of 0.999 or better. Reproducibility of the method was established for nine replicate analyses of a solution of Knox gelatin. Relative standard deviations were 1–7% for all amino acids except methionine, which was 24%. Methionine is unstable under conditions of acid hydrolysis unless a preservative is present.
3 APPENDIX 3: TROUBLESHOOTING
Although the ECF method has proven to be extremely reliable, a certain amount of routine troubleshooting was needed to obtain consistent results on a daily basis. It is our experience that, when properly maintained, a column should last for more than 300 injections.
The primary concern was the effect of capillary column and liner activity on peak areas and detector response factors. Reaction of the fused silica and quartz surfaces with water and oxygen produces silanol groups that can undergo hydrogen-bonding with certain analytes. Hydroxy-substituted amino acid derivatives (serine, threonine, and hydroxyproline) were most severely affected by activity because ECF does not derivatize every active hydrogen. Activity was minimized by: (1) installation of oxygen and moisture traps in the carrier gas line; (2) carefully separating the chloroform layer from the aqueous layer and drying the chloroform layer over sodium sulfate to remove water from the injection volume; (3) changing the inlet liner every 30–50 injections to keep semivolatile active contaminants out of the column; (4) baking the column for 4 hours at 250�C if peak tailing is observed.
Peak resolution is another consideration in maintaining optimum system performance. In developing a temperature program for a particular column the problem of “tunable selectivity” is encountered (the phenomenon in which elution order changes with heating rate). This effect is evident for the triplet of methionine, serine, and glutamic acid in figure 2. As a column ages, the resolution between serine and glutamic acid decreases. Improved resolution may be obtained by increasing the heating rate in the temperature program by 2�C or more. Of course, this effect cannot be maintained indefinitely; column replacement will be necessary when improvement in resolution between serine and glutamic acid is obtained at the expense of a decrease in resolution between methionine and serine.
REFERENCES
Bergquist, D.. 1981. Eggs. In Kirk-Othmer encyclopedia of chemical technology, 3d ed.8:429–45.
Collings, T. J., and F. J.Young. 1976. Improvements in some tests and techniques in photograph conservation. Studies in Conservation21:79–84.
Grzywacz, C. M.1994. Identification of proteinaceous binding media in paintings by amino acid analysis using 9-fluorenylmethyl chloroformate derivatization and reversed-phase high-performance liquid chromatography. Journal of Chromatography A676(1): 177–83.
Hall, C. W.1981. Milk and milk products. In Kirk-Othmer encyclopedia of chemical technology, 3d ed.8:522–70.
Halpine, S.1992. Amino acid analysis of proteinaceous media from Cosimo Tura's The Annunciation with Saint Francis and Saint Louis of Toulouse. Studies in Conservation37:22–38.
Hamilton, P. B.1965. Amino-acids on hands. Nature205:284–85.
Hušek, P.1991. Rapid derivatization and gas chromatographic determination of amino acids. Journal of Chromatography552:289–99.
Karpowicz, A.1981. Ageing and deterioration of proteinaceous media. Studies in Conservation26:153–60.
Keck, S., and T.PetersJr.1969. Identification of protein-containing paint media by quantitative amino acid analysis. Studies in Conservation14:75–82.
K�hn, H.1986. Conservation and restoration of works of art and antiquities. London: Butterworths. 1:157–67.
Masschelein-Kleiner, L.1974. An improved method for the thin-layer chromatography of media in tempera paintings. Studies in Conservation19:207–11.
Masschelein-Kleiner, L.1976. Contribution to the study of aged proteinaceous media. In Conservation and restoration of pictorial art, ed.N. S.Brommelle and P.Smith. London: IIC–Butterworths. 84–88.
Mills, J. S., and R.White.1978. Organic analysis in the arts: Some further paint medium analyses. National Gallery (London)Technical Bulletin2:71–76.
Mills, J. S., and R.White. 1987. The organic chemistry of museum objects. London: Butterworths. 73–82.
Pancella, R., and R.Bart. 1989. Identification des liants organiques dans les couches picturales par chromatographie en phase gazeuse. Zeitschrift f�r Kunsttechnologie3(3): 101–11.
Pickering, M. V.1989. Ion-exchange chromatography of free amino acids. LC/GC7(6): 484–90.
Pickering, M. V., and P.Newton. 1990. Amino acid hydrolysis: Old problems, new solutions. LC/GC8(10): 778–81.
Rom�o, P.M.S., A. M.Alarc�o, and C.A.N.Viana. 1990. Human saliva as a cleaning agent for dirty surfaces. Studies in Conservation35:153–55.
Ronca, F.1994. Protein determination in polychromed stone sculptures, stuccoes and gesso grounds. Studies in Conservation39:107–20.
Sack, S. P., F. C.Tahk, and T.Peters. 1981. A technical examination of an ancient Egyptian painting on canvas. Studies in Conservation26:15–23.
Schilling, M., and H.Khanjian. Gas chromatographic analysis of amino acids as ethyl chloroformate derivatives. Part 2, Effects of pigments and accelerated aging on the identification of proteinaceous binding media. In preparation.
Sharon, N.1975. Complex carbohydrates: Their chemistry, biosynthesis, and functions. Reading, Mass.: Addison-Wesley.
Sinkai, T., and R.Sugisita. 1990. Identification of protein-containing binding media and adhesives in works of art by amino acid analysis. Journal of Archaeological Science of Japan35:1–12.
Striegel, M.1994. Methods in scientific examination of works of art: Thin-layer chromatography. Course notebook. Getty Conservation Institute, Marina del Rey, Calif.
Stulik, D. C., C. M. G.Druzik, M. R.Derrick, and J. M.Landry. 1991. Standard materials for analysis of binding media and GCI binding media library. AIC Abstracts, American Institute for Conservation 19th Annual Meeting, Albuquerque. Washington, D.C.: AIC.
Stulik, D., and H.Florsheim. 1992. Binding media identification in painted ethnographic objects. Journal of the American Institute for Conservation31:275–88.
Von Endt, D., and M.Baker. 1991. The chemistry of filled animal glue systems. In Gilded wood: Conservation and history, ed.D.Bigelow et al. Madison, Conn.: Sound View Press. 155–62.
White, R.1984. The characterization of proteinaceous binders in art objects. National Gallery (London) Technical Bulletin8:5–14.
SOURCES OF MATERIALSEthyl chloroformate 18,589–2:Aldrich Chemical Co. Inc., 1001 W. St. Paul Ave., Milwaukee, Wisc. 53201 C32 Microbalance with remote weighing unitCahn Instruments, Inc., 16207 S. Carmenita Rd., Cerritos, Calif. 90701 HP 5890 Gas Chromatography with HP 5895 GC ChemStation; HP-INNOWAX capillary column, 0.25 mm ID, 0.25 μm film thickness, 15M length, 19091N–131Hewlett Packard Co., 2850 Centerville Rd., Wilmington, Del. 19808 DB-WAX capillary column, 0.25 mm ID, 0.25 μm film thickness, 15M length, 122–7012; DB–1701 capillary column, 0.25 mm ID, 0.25 μm film thickness, 15M length, 122–1312J and W Scientific, 91 Blue Ravine Rd., Folsom, Calif. 95630–4714 OV–1701 capillary column, 0.25 mm ID, 0.25, μm film thickness, 15 M length, 415–2502Ohio Valley Specialty Chemical, 115 Industry Rd., Marietta, Ohio 45750 6N hydrochloric acid–sequanal-grade 24309; HPLC-grade water 51140 pyridine 27530; 25 ml screw-top septum vials 13074; Mininert valves for 25 ml screw-top vials 10130Pierce Chemical Co., 3747 N. Meridian Rd., Rockford, Ill. 61105–0117, 2, 20 and 100 μl Pipetman micropipettorsRainin Instrument Co., Inc., P. O. Box 4026, Woburn, Mass. 01888–4026 AA calibration standard A2161Sigma Chemical Co., 3050 Spruce St., St. Louis, Mo. 63178 anhydrous 200 proof ethanol E1028Spectrum Chemical Mfg. Corp., 14422 S. San Pedro St., Gardena, Calif. 90248
AUTHOR INFORMATION
MICHAEL R. SCHILLING earned his B. S. (1983) and M.S. (1990) in chemistry from California State Polytechnic University, Pomona. He has worked at the Getty Conservation Institute since 1983 and presently holds the position of associate scientist. He has been active in the examination of painted museum objects, pigment identification, binding medium analysis, and analysis of volatile organic compounds in the museum environment. He has also been involved in a number of special GCI collaborative projects in Egypt, China, and Israel. Address: Getty Conservation Institute, Scientific Program, 4503 Glencoe Ave., Marina del Rey, Calif. 90292.
HERANT P. KHANJIAN received his B.A. in chemistry from California State University, Northridge. His research interests involve the detection and identification of binding media found in art objects using gas chromatography and infrared spectroscopy. Address as for Schilling.
LUIZ A. C. SOUZA received his B.S. (1986) and M.Sc. (1991) in chemistry from the Federal University of Minas Gerais, Brazil. In 1987–88 he held an internship at the Scientific Department of the Royal Institute of Cultural Properties (IRPA) in Brussels, Belgium. Since 1989 he has been teaching and researching at the Center for Conservation and Restoration of Cultural Movable Properties, Federal University of Minas Gerais, Brazil. His research interests are preventive conservation in the tropics and the history of techniques and technology of paintings and polychromed sculptures. During 1992 and 1993 he had a research fellowship in the Scientific Program at the Getty Conservation Institute, working with scientific analysis and identification of materials of baroque and rococo polychromed sculptures from Minas Gerais, Brazil. Address: Luiz A. C. Souza, CECOR, EBA, Universidade Federal de Minas Gerais, 31270–901, Belo Horizonte, MG, Brasil.
Section Index |