CONTROLS ON STONE TEMPERATURES AND THE BENEFITS OF INTERDISCIPLINARY EXCHANGE
J. P. MCGREEVY, P. A. WARKE, & B. J. SMITH
ABSTRACT—ABSTRACT—Data derived from a series of field and laboratory studies of the influence of albedo and thermal conductivity on stone temperatures are reported. The data indicate the complexity of surface and subsurface temperature response characteristics of different stone types exposed to the same conditions. They also highlight the influence of albedo and thermal conductivity on microenvironmental conditions at the rock-air interface. These conditions have significant implications for the nature and rate of weathering activity and may, over time, affect any treatments applied to stone surfaces. Although the studies reviewed were carried out within the subject area of geomorphology, the data reported and the implications for stone weathering arising from them may be of some relevance to the conservation science perspective on deterioration of contemporary, historical, and archaeological stonework.
TITRE—Le contr�le de la temp�rature de la pierre et les b�n�fices de l'�change interdisciplinaire. R�SUM�—Cet article pr�sente les donn�es obtenues lors d'une s�rie d'�tudes en laboratoire et de tests pratiques afin de d�terminer l'influence de l'alb�do et de la conductivit� thermique sur la temp�rature de la pierre. Ces donn�es indiquent la complexit� des modes de r�action de la temp�rature en surface et sous-jacente de diff�rents types de pierres expos�es � des conditions identiques. Elles mettent �galement en valeur l'influence de l'alb�do et de la conductivit� thermique sur les conditions micro-climatiques � l'interface pierre-air ambiant. Ces conditions jouent un r�le important quant au type et � la rapidit� de l'�rosion de la pierre due aux agents atmosph�riques, et peuvent donc � long terme affecter le r�sultat des traitements de surface de la pierre. Bien que ces �tudes aient �t� conduites dans un contexte g�omorphologique, les donn�es obtenues sur l'�rosion de la pierre due aux agents atmosph�riques peuvent �tre pertinentes dans le domaine des sciences de la restauration, en ce qui concerne la d�t�rioration des ouvrages de ma�onnerie historiques, arch�ologiques et contemporains.
TITULO—Beneficios de intercambio interdisciplinario en la medida de temperatura de piedras. RESUMEN—Se informa sobre valores obtenidos de una serie de estudios in situ y de laboratorio sobre la influencia de la conductividad t�rmica y la fracci�n de luz difusa reflejada de la superficie de piedras. Los valores muestran una respuesta t�rmica compleja tanto superficial como sub-superficial de los distintos tipos de piedras expuestos en id�nticas condiciones. Tambi�n demuestran la influencia de la conductividad t�rmica y la fracci�n de luz difusa reflejada sobre las condiciones microambientales en la interfase roca – aire. Estas condiciones tiene un importante efecto sobre el tipo y velocidad de intemperismo (deterioro), y puede, a la larga, afectar a los tratamientos aplicados a superficies de piedras. A pesar que los estudios rese�ados se llevaron a cabo dentro del �rea de la geomorfolog�a, la informaci�n obtenida y las implicaciones para el deterioro de la piedra pueden ser de relevancia para la conservaci�n de la piedra en estructuras contemporarias, hist�riocas y arquel�gicas.
1 1. INTRODUCTION
A number of recent studies at various nationally and internationally important archaeological sites have used microenvironmental data to infer causes of stone deterioration as a basis for formulating conservation strategies (Thorn 1994; Maekawa et al. 1995; Maekawa and Agnew 1996; Thorn and Piper 1996). Underpinning this approach is the view that at any individual site or monument, identification of probable causes of deterioration “demands a more exact knowledge of the environment at the site as well as responses of the monument materials to the environment” (Maekawa et al. 1995, 886).
Because temperature is one of the most significant factors in rock breakdown, operating indirectly through its control on moisture movement and processes such as salt weathering and freeze-thaw and, possibly, directly through “insolation weathering” (structural deterioration of stone by repeated thermally induced expansion and contraction of surface material), attention has inevitably been directed toward stone temperature data in both natural and man-made structures. In the above-mentioned studies, interpretation and discussion of stone temperature data have usually been couched in terms of relationships among such parameters as temperature maxima, minima, ranges, and gradients and what are essentially positional influences such as orientation and inclination of stone surfaces. Occasionally, when more than one stone type is present at the same site, reference has been made to the possibility that, because of differences in thermal properties, different stone types can display dissimilar temperature regimes under similar conditions of exposure (Maekawa et al. 1995). However, the significance of such variations for stone durability is still not fully understood but may have implications for those directly concerned with conservation of stone artifacts and any associated decoration exposed to subaerial conditions.
This article reports a series of field and laboratory experiments that are used to explore the influence of the thermal properties of albedo and conductivity on stone temperature maxima and gradients under a range of ambient conditions. These experiments have been carried out within the subject area of geomorphology, a discipline that, although not specifically related to stone conservation, is, through its concentration on the understanding of weathering processes, of direct relevance to it. Finally, the reported findings are offered in response to a recent call for more interdisciplinary dialogue in matters of common interest (Price 1996).
2 2. FIELD STUDIES
2.1 2.1 BACKGROUND
Over the past 20 years, researchers based at the School of Geography, the Queen's University of Belfast, have conducted various field studies aimed at gathering stone temperature data from relatively extreme climatic regions: Morocco (Smith 1977; Kerr et al. 1984); Iceland (Douglas et al. 1983); Karakoram Himalayas (Whalley et al. 1984); Tenerife (Jenkins and Smith 1990); and Death Valley, California (Warke 1994; Warke and Smith 1998).
The general purpose of these studies, which complement work carried out by other geomorphologists (see reviews by Smith 1994 and Goudie and Viles 1997), has been to improve understanding of the temperature conditions under which stone weathering occurs. Notwithstanding the intricacies of microclimate (Geiger 1965), it is clear that there are problems in using standard meteorological data on air temperatures, often recorded at some distance from sites of interest, to infer causes of decay. Specifically, the data collected have been used to guide the design of laboratory simulation experiments and to examine the extent to which albedo and thermal conductivity, in particular, influence the temperature regimes experienced by different stone types. It is these specific aims and the information arising from them that may be of relevance to those interested in the deterioration and conservation of monumental stonework.
2.2 2.2 METHODS
2.2.1 2.2.1 Materials
A number of stone types were used in the field studies and laboratory investigations (table 1). These were chosen to represent a range of physical and mineralogical characteristics that allow the investigation of rock property controls on surface and near-surface temperatures. In particular, the rocks display a variety of colors (albedo) ranging from very dark gray basalt to white chalk and marble, with reddish brown and pale brown sandstones, gray granite, and grayish white limestone lying between these extremes.
Table 1. Some Genearl Characteristics of Stone Types Used for Data Collection
Stone Type | Munsell Color Notation (dry) and Color Description | Albedo(%) | Porosity(%) | Average Ultrasound Pulse Velocity (m sec−1 | Thermal Conductivitya (Wm−1 K−1) | General Description |
Antrim basalt | 2.5Y N3/0(very dark gray) | 12 | 1.83 | 6,155 | 0.96 | tertiary olivine-rich, fine-grained basalt with some microfracture development |
Mourne granite | 5YR 6/1 (gray) | 18 | 1.85 | 4,425 | 1.65 | tertiary quartzose, coarse-grained granite with tightly interlocked crystal structure |
Baumberger sandstone | 10YR 7/2 (7/3) (light gray, very pale brown) | NA | 21.40 | 3,004 | N/A | Cretaceous fine-grained clacareous sandstone |
Dunhouse sandstone | 10YR 7/2 (7/3) (light gray, very pale brown) | NA | 18.10b | 2,460 | N/A | carboniferous noncalcareous fine- to medium-grained sandstone with interstitial kaolinite deposits |
Scrabo sandstone(c) | 2.5YR 5/4 (dull reddish brown, but color can be extremely variable) | 12 | 14.80–21.24 (extremely variable) | 2,274 | 1.05 | Triassic noncalcareous, fine to medium-grained sandstone with bedding planes, clay inclusions and lenses (smectites) and variable packing of constituent grains |
Portland limestone | 2.5YR 8/1 (grayish white) White | NA | 17.90 | 3,978 | 1.53 | Jurassic oolitic limestone with bimodal pore size distributions |
Pentellic marble | White (no suitable Munsell notaion) | NA | 0.18 | 5,097 | N/A | Jurassic dense white marble |
Antrim chalk | 5YR 8/1(white) | 25 | 8.27 | 5,192 | 1.72 | Cretaceous compact chalk with tightly interlocking blocky micritic crystals |
NA = not available
a aAlthough specific thermal conductivity values were not obtainable for all eight rock types, it was possible to assign relative thermal conductivity positions on the basis of simultaneous surface-subsurface temperature differences arising from exposure to direct heating under laboratory conditions with the thermal conductivity of Antrim basalt < Scrabo sandstone < Baumberger sandstone < Portland limestone < Mourne granite < Pentellic marble ≤ Dunhouse sandstone < Antrim chalk. This ordering corresponds resonably closely with known thermal conductivity values.
b bLeary 1986
(c) cValues given may not be representative of Scrabo sandstone in general because of its structural and mineralogical variability.
|
2.2.2 2.2.2 Temperature Measurements and Conditions of Exposure
For two reasons, the data presented focus on hot climates (Morocco and Death Valley, California): first, because of the interest in stone deterioration on buildings and monuments located in regions that, in terms of temperatures, are climatically similar (for example, southern Europe, North Africa, the Middle East, Central America), and second, because they relate to the laboratory studies described later.
Comparative investigation of the responses of different stone types to heating and cooling under natural exposure conditions is often constrained by little or no variation in geology at study sites, by the fact that valid comparisons rely on stone surfaces being similarly orientated and inclined, and by the practical difficulties of drilling into stone to allow subsurface temperature measurements. To overcome these obstacles, we have resorted to the use of precut and predrilled blocks, normally 5–7 cm cubes of a range of stone types embedded in expanded polystyrene. The polystyrene jackets promote unidirectional heating and cooling that is characteristic of larger surfaces in natural situations. Because our interests are related primarily to surface and near-surface weathering phenomena, depths of temperature measurement were not great, varying between 2.5 cm and 6.0 cm (fig. 1).
Fig. 1.
Detail of insulated blocks and position of thermistors used in collection of temperature data from Death Valley and laboratory studies, and Southeast Morocco
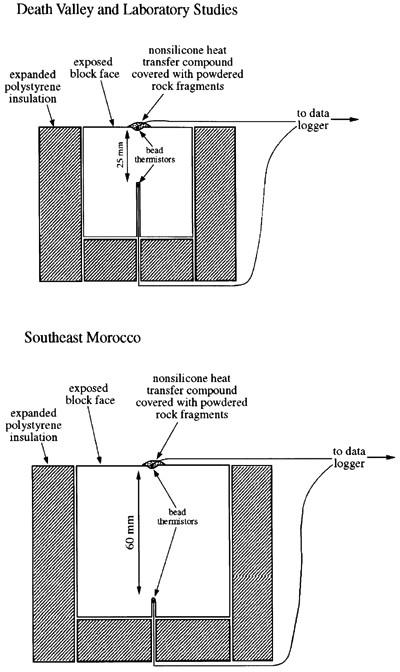 |
Temperatures were measured using various instruments, usually Grant Instrument Squirrel data loggers and bead thermistors. For surface temperature measurements, shallow holes (2–3 mm) were drilled on the exposed sample surface. A bead thermistor was then placed in the hollow and fixed to the stone surface using a nonsilicone heat conducting compound (Heat Sink Compound). The surface of this was then well covered with powdered fragments from the same stone type in order to maintain a uniform surface albedo and prevent any disparity in surface heating. Subsurface temperatures were measured by drilling up from the base of the block to a point 6 cm from the surface in the case of the samples used in Southeast Morocco and 2.5 cm for the blocks used in Death Valley and the laboratory studies.
2.3 2.3 FIELD RESULTS
2.3.1 2.3.1 Southeast Morocco
Figure. 2 depicts surface and subsurface temperatures experienced by blocks of Antrim chalk (white limestone), Mourne granite, and Scrabo sandstone exposed at a site in Southeast Morocco. There are distinct differences between the maximum surface temperatures for each stone type and also between the differences in surface and subsurface maxima. The values for the latter are 0.9�, 1.6�, and 3.0�C for chalk, granite, and sandstone, respectively. These are not, however, the maximum internal temperature differences that occur during the heating phase, which are 3.5�, 4.3�, and 5.4�C, respectively.
Fig. 2.
Surface and subsurface (6 cm depth) temperature measurements for Antrim chalk, Mourne granite, and Scarbo sandstone blocks exposed to natural hot desert conditions in Southeast Morocco
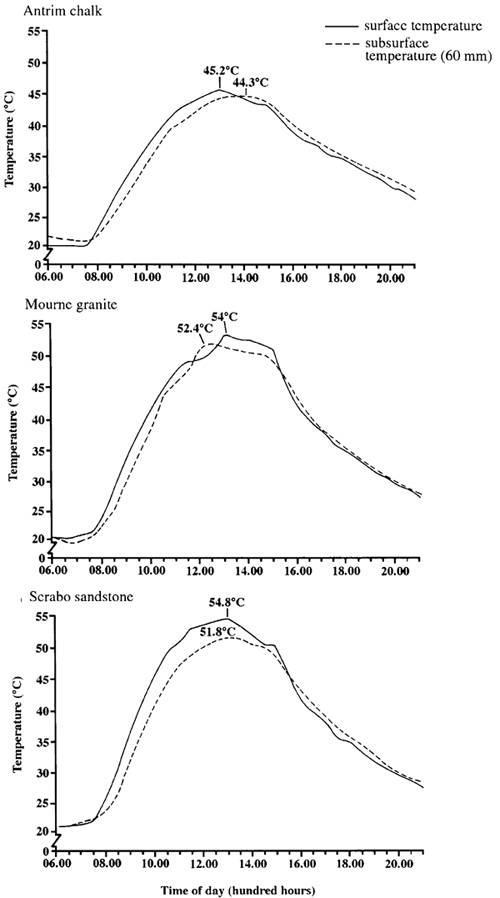 |
Primarily these data can be used to illustrate the control exerted by thermal conductivity (see table 1) on subsurface heating, whereby the stone with the highest thermal conductivity value (chalk) experiences the lowest internal temperature gradient, reflecting enhanced heat transference to depth in comparison to the other stone types. This control is further reflected by the granite, which has a lower maximum surface temperature than Scrabo sandstone but a higher temperature at depth. Absolute surface temperature, however, appears to be controlled more by a combination of surface albedo (coloration) and thermal conductivity. Intuitively, it would be expected that favorable combinations of these would enhance surface heating. Thus a combination of a dark surface and low thermal conductivity should be more conducive to the creation of high surface temperatures than the presence of only one of these characteristics. This speculation is borne out by the data: Scrabo sandstone, with both the lowest albedo and thermal conductivity, attained the highest surface temperature while chalk, with the highest albedo and thermal conductivity, reached a much lower maximum surface temperature. Granite, with intermediate values for albedo and thermal conductivity, exhibited a maximum surface temperature between these two extremes.
2.3.2 2.3.2 Death Valley
The Mourne granite from the Moroccan study was combined with three other stone types—Antrim basalt, Portland limestone, and Dunhouse sandstone—in a similar investigation at a site in Death Valley, California. Again, considerable differences among the lithologies are evident (fig. 3). The effect of dark surface coloration combined with low thermal conductivity is dramatically illustrated by the surface temperatures of the basalt sample (maximum 72.65�C). The contrast with the other stone types, particularly Portland limestone with its light-colored surface and much higher thermal conductivity, is marked. As with the Moroccan data, internal temperature gradients reflect the influence of thermal conductivity, the one varying inversely with the other.
Fig. 3.
Surface and subsurface temperature characteristics of four lithologies exposed to natural hot desert conditions in Death Valley National Monument, California, August 3, 1992. Reprinted from Warke and Smith 1998, with permission from Elsevier Science.
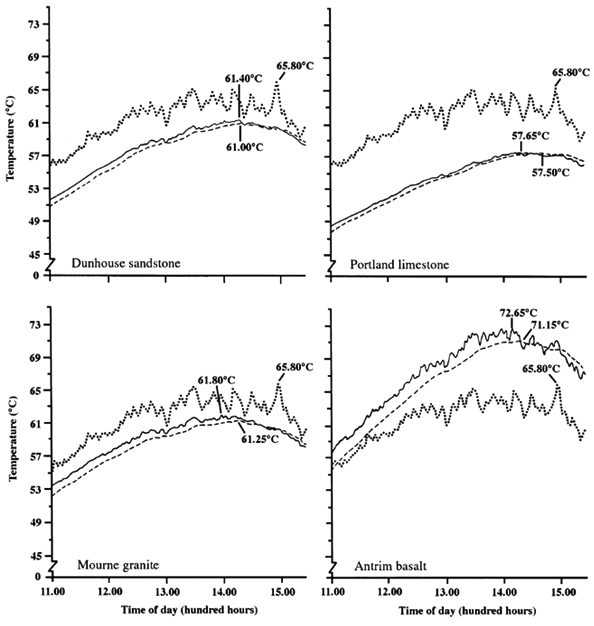 |
3 3. LABORATORY STUDIES
3.1 3.1 BACKGROUNG
The laboratory studies described in the following sections both parallel and extend those conducted in the field by examining, under controlled conditions, the effects of surface color change (staining) on stone temperature regimes (Warke et al. 1996) and the effects of direct and indirect heating on different stone types (Warke and Smith 1998). The prompt for examining the former was an interest in the soiling of stone surfaces that are common on buildings and monuments in polluted urban environments and that are cited as being both a cause and an effect of weathering (Smith and Magee 1990). With regard to the latter, it is invariably the case in laboratory-based durability tests (Ross and Butlin 1989) that stone samples are heated and cooled using oven-based (i.e., indirect) methods whereby the temperature of samples is determined solely by the temperature of the surrounding air. This form of temperature control neglects the potential influence of albedo, with the result that all samples are likely to experience the same temperature regime and thus not experience the surface/subsurface temperature differences that may be produced under natural conditions. It was felt, therefore, that the extent to which this approach failed to discriminate among different stone types would be worthy of quantification.
3.2 3.2 METHODS
3.2.1 3.2.1 Experimental Design
The equipment used to examine the effect of staining on stone surface temperature characteristics was specifically designed and constructed to simulate direct heating of stone samples as well as repeated short-term temperature fluctuations associated with, for example, passing cloud cover (fig. 4). It comprises a large drum 75 cm in diameter and 100 cm deep. Three ports located at regular intervals around the circumference of the drum allow access to the interior, and three infrared lamps fixed over the drum provide the heat source. Interruptions to this heat source are achieved by a rotating semi-circular blade driven by a “stepping motor” that can be set to revolve at speeds ranging from 1 rev/8 hours to 16 rev/hour. When, for example, the blade is set to rotate at 2 rev/hour, any stone samples beneath it will experience two shade episodes, each of 15 minutes duration, in every hour. When all three lamps are on, they create within the drum a relatively stable air temperature of 40�C, henceforth referred to as “warm air” conditions that are typical of Mediterranean summer regimes.
Fig. 4.
Diagram of a detail of circular drum used in simulation experiments
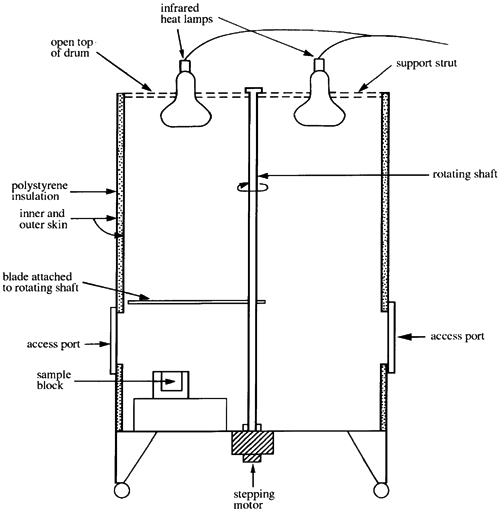 |
Direct and indirect heating effects were investigated using an environmental chamber (Design Environmental 990–20H Chamber) modified by the addition of an infrared lamp positioned approximately 40 cm above the stone samples. As with the drum, short-term temperature fluctuations were also examined, the effect being achieved within the chamber by using a timing control switch to turn the lamp on and off at 15 minute intervals.
Temperatures were measured at 1 minute intervals, and in all experiments bead thermistors and a 12 bit Grant Squirrel data logger were used.
3.2.2 3.2.2 Materials
Stone samples used in the laboratory investigations were prepared as described in section 2.2.1 and depicted in figure 1. The four types selected for the Death Valley field study (Antrim basalt, Mourne granite, Dunhouse sandstone, and Portland limestone) were used in the study of direct and indirect heating effects, while, to investigate the influence of surface soiling on temperature regimes Pentellic marble, Baumberger sandstone, Dunhouse sandstone, and Portland limestone were used. Soiling of test specimens was simulated by fixing a thin dusting of either coal or oil fly ash onto stone surfaces using an aerosol adhesive, producing, respectively, light gray (Munsell notation 10YR 7/2) and black (10YR 2/1) coloration.
3.3 3.3 LABORATORY RESULTS
3.3.1 3.3.1 Soiling as an Influence on Temperature Characteristics
Data presented in figure 5 and table 2 show the differences among the four stone types used in the soiling experiment under warm air conditions. When clean, all four samples present similarly light-colored (high albedo) surfaces, but differences in thermal response characteristics indicate the underlying influence of thermal conductivity. Baumberger sandstone and Portland limestone reached the highest surface temperatures, with the former displaying the greatest surface temperature decrease during each 15 minute period of shade. This finding may reflect a low thermal conductivity and, possibly, poor heat storage in surface layers from where it is rapidly lost once incident radiation is interrupted. Simultaneous surface and subsurface temperature measurements tend to support this relationship, as Baumberger sandstone exhibited the greatest surface/subsurface differences, while Dunhouse sandstone and Pentellic marble showed comparable surface characteristics under these warm air conditions.
Fig. 5.
Surface and subsurface temperature data from insulated clean and soiled stone samples exposed to heating in the circular drum under warm air conditions (Warke et al. 1996) �John Wiley and Sons Limited. Reproduced with permission
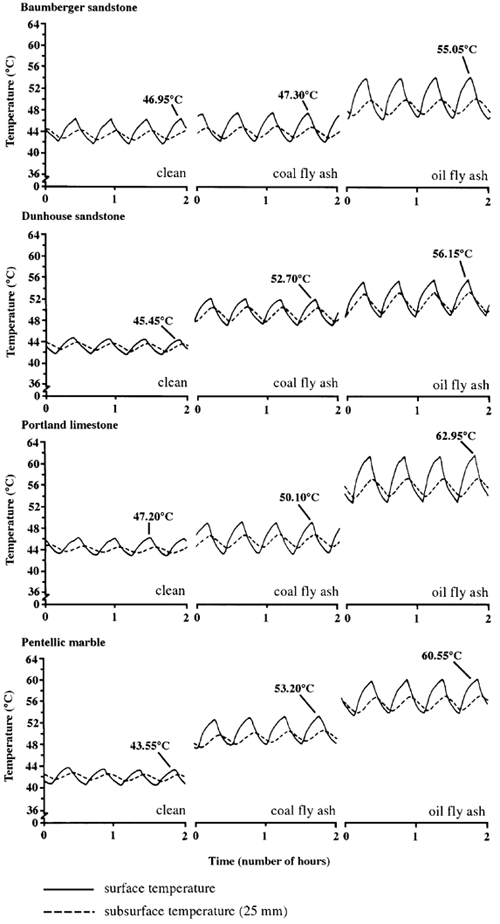 |
Table 2. Rates of Surface Temperature Change for Clean and Soiled Surfaces under Warm Air Conditions
BAUMBERGER SANDSTONE |
| Surface Temp. (�C) | TC after 1st 5 mins (�C) | Rate of TC (�C/mins) | TC after 2d 5 mins (�C) | Rate of TC (�C/min) | TC after 3d 5 mins (�C) | Rate of TC (�C/min) | Total TC (�C) | Avg. TC per 15 min cycle (�C/min) |
Clean surface | 46.95 | 2.55 | 0.51 | 1.35 | 0.27 | 1.15 | 0.23 | 5.05 | 0.34 |
Warm air, coal fly ash | 47.30 | 2.60 | 0.52 | 1.70 | 0.34 | 0.90 | 0.18 | 5.20 | 0.35 |
Warm air, oil fly ash | 55.05 | 3.55 | 0.71 | 2.70 | 0.54 | 1.30 | 0.26 | 7.55 | 0.50 |
DUNHOUSE SANDSTONE |
Clean surface | 45.5 | 1.55 | 0.31 | 1.00 | 0.20 | 0.60 | 0.12 | 3.15 | 0.21 |
Warm air, coal fly | 52.70 | 2.65 | 0.53 | 1.40 | 0.28 | 0.90 | 0.18 | 4.95 | 0.33 |
Warm air, oil fly ash | 56.15 | 8.85 | 0.77 | 1.65 | 0.33 | 0.10 | 0.22 | 6.60 | 0.44 |
PORTLAND LIMESTONE |
Clean surface | 47.20 | 1.15 | 0.23 | 1.10 | 0.22 | 0.85 | 0.17 | 3.10 | 0.21 |
Warm air, coal fly | 50.10 | 3/05 | 0.61 | 1.70 | 0.34 | 0.70 | 0.14 | 5.45 | 0.36 |
Warm air, oil fly ash | 62.95 | 4.85 | 0.97 | 2.35 | 0.47 | 3.15 | 0.63 | 10.35 | 0.69 |
PENTELLIC MARBLE |
Clean surface | 43.55 | 1.35 | 0.27 | 1.10 | 0.22 | 0.65 | 0.13 | 3.10 | 0.21 |
Warm air, coal fly | 53.20 | 2.75 | 0.55 | 1.30 | 0.26 | 2.00 | 0.40 | 6.05 | 0.40 |
Warm air, oil fly ash | 60.55 | 3.60 | 0.72 | 2.45 | 0.49 | 1.85 | 0.37 | 7.90 | 0.53 |
TC = temperature change
|
Surface soiling with both coal and oil fly ash increased the surface temperatures of all four stone types, particularly Portland limestone and Pentellic marble. Portland limestone treated with oil fly ash exhibited surface temperatures more than 15�C higher, increasing during each 15 minute period from 0.21�C min−1 to 0.69�C min−1. In all cases, surface soiling, especially with oil fly ash, increased the magnitude of surface temperature change during each 15 minute period. When shaded, surface temperature of Portland limestone decreased most rapidly, followed by Baumberger sandstone, Dunhouse sandstone, and Pentellic marble. In all cases, approximately half the total temperature decrease occurred during the first 5 minutes of shade. Surface soiling increased radiant heat absorption, raising temperatures well above ambient air temperature such that, when the heat source was interrupted, heat was rapidly lost to the cooler surrounding air.
From the above results and observations, it would appear that soiling affects surface temperatures of light-colored stone in several ways. First, and most obviously, it raises surface temperatures. Second, under conditions of direct heating, it increases the rate of temperature decrease when the heat source is interrupted, especially when thermal conductivity is low. Finally, through a combination of these effects, it enhances the characteristic asymmetrical pattern of surface heating and cooling. Increased rates of heat loss/gain must, in turn, increase the magnitude of thermal stresses developed between individual anisotropic mineral grains and between the surface and subsurface parts of the stone.
An examination of temperature changes with depth reveals that Baumberger sandstone exhibited the largest surface/subsurface temperature differences and the highest average temperature gradient with depth (table 3). This finding suggests that it does not readily conduct heat from surface to subsurface layers. Portland limestone displayed the next steepest gradient, while the data from Dunhouse sandstone and Pentellic marble show reduced surface/subsurface temperature gradients, suggesting more transference of heat from surface to substrate.
Table 3. Rate of Temperature Change with Depth on Clean and Soiled Surfaces under Warm Air Conditions
BAUMBERGER SANDSTONE |
| Max Surf. Temp. (�C) | Simultaneous Subsurf. Temp. (2.5 cm) | Diff. (�C) | Rate of Decrease (�C/cm) | Min. Surf. Temp. (�C) | Temp. Range per Cycle (�C) |
Clean surface | 46.95 | 44.15 | 2.80 | 1.12 | 41.90 | 5.05 |
Warm air, coal fly | 47.30 | 44.35 | 2.95 | 1.18 | 42.10 | 5.20 |
Warm air, oil fly ash | 55.05 | 50.30 | 4.75 | 1.90 | 47.50 | 7.55 |
DUNHOUSE SANDSTONE |
Clean surface | 45.45 | 44.35 | 1.10 | 0.44 | 42.30 | 3.15 |
Warm air, coal fly ash | 52.70 | 50.95 | 1.75 | 0.70 | 47.75 | 4.95 |
Warm air, oil fly ash | 56.15 | 54.05 | 2.10 | 0.84 | 49.55 | 6.60 |
PORTLAND LIMESTONE |
Clean surface | 47.20 | 45.25 | 1.95 | 0.78 | 44.10 | 3.10 |
Warm air, coal fly | 50.10 | 47.55 | 2.55 | 1.02 | 44.65 | 5.45 |
Warm air, oil fly ash | 62.55 | 58.20 | 4.75 | 1.90 | 52.60 | 10.35 |
PENTELLIC MARBLE |
Clean surface | 43.55 | 42.25 | 1.30 | 0.52 | 40.45 | 3.10 |
Warm air, coal fly ash | 53.20 | 50.35 | 2.85 | 1.14 | 47.15 | 6.05 |
Warm air, oil fly ash | 60.55 | 57.00 | 3.55 | 1.42 | 52.65 | 7.90 |
Because soiling, especially with oil fly ash, raises surface temperatures, it also increases temperature gradients within the specimens, particularly in Baumberger sandstone and Portland limestone. Soiling (oil fly ash) increased temperature gradients within these stone types by 70% and 140%, respectively.
The evidence suggests, therefore, that surface soiling of light-colored stone with a low thermal conductivity raises surface temperatures and increases surface/subsurface temperature gradients. Inevitably, these increases must raise thermal stress between surface and subsurface layers and affect microenvironmental conditions at the stone-air interface, increasing the complexity of interactions between weathering activity and stone response in this zone, particularly where soiling patterns are complex and soiled and unsoiled surfaces are adjacent.
3.3.2 3.3.2 Direct and Indirect Heating as an Influence on Temperature Regimes
Comparison of the field data described earlier (see fig. 3) with the results of oven-based (i.e., indirect) heating of specimens of the same four stone types in an environmental chamber (fig. 6) is informative. It is clear that the variations evident under natural conditions of exposure are not replicated by a heating method that results in the pattern of temperature change of the specimens mirroring that of the surrounding air—a drawback given the potential for variation as a result of thermal properties, especially albedo. The relative differences among the stone types encountered under natural conditions can, however, be replicated by using a direct infrared heat source (fig. 7). In this way, the effects of differing thermal properties become evident, with basalt attaining the highest surface and subsurface temperatures and Portland limestone the lowest, despite exposure to the same conditions.
Fig. 6.
Surface and subsurface temperature characteristics of four lithologies exposed to indirect (air) heating in an environmental chamber under simulated hot desert conditions. Reprinted from Warke and Smith 1998, with permission from Elsevier Science.
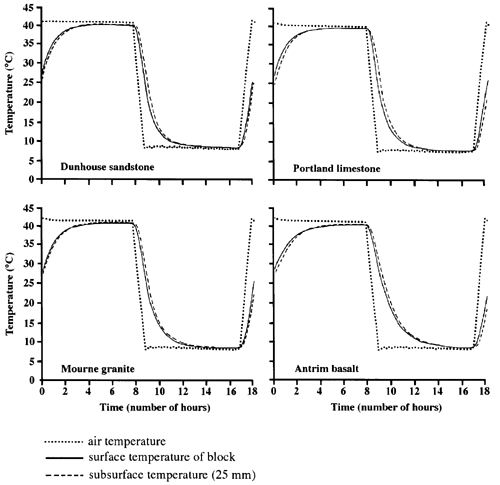 |
Fig. 7.
Surface and subsurface temperature characteristics of four lithologies exposed to direct (infrared) and indirect (air) heating in an environmental chamber under simulated hot desert conditions. Reprinted from Warke and Smith 1998, with permission from Elsevier Science.
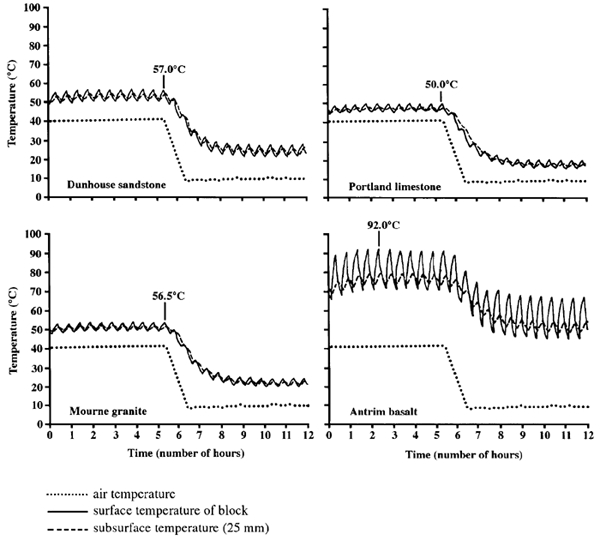 |
4 4. DISCUSSION AND IMPLICATIONS
A fundamental point that emerges from the data presented here is that albedo and thermal conductivity can exert a significant influence on maximum stone surface temperatures and surface-subsurface temperature gradients. While this effect seems obvious, relevant empirical evidence is rare, and such properties are not generally taken into account in considerations of stone weathering under either field or laboratory conditions.
In terms of conservation studies, the data presented have several implications. First, microenvironmental conditions experienced at and just below stone surfaces can be more variable and more, or indeed less, extreme than macroenvironmental data, particularly air temperature, might suggest. Consequently, inferences regarding the nature and potential effectiveness of stone deterioration processes drawn solely from meteorological observations should be guarded. The view that detailed environmental monitoring should inform the development of conservation programs and management strategies at cultural sites (Thorn 1994; Thorn and Piper 1996) is reinforced by the data reported here. Particular care should be taken not to generalize where more than one stone type is present at sites under investigation.
Second, results from the direct and indirect heating experiments raise questions concerning the usefulness of laboratory-based stone weathering simulations and durability tests, not only of the stone itself but of any surface treatments applied to the stone surface. Invariably, indirect heating methods are used and result in test specimens' being cycled through “lithologically indiscriminate” temperature regimes. Stone types with a high albedo (such as the Portland limestone reported here) are, therefore, likely to be subjected to high surface and subsurface temperatures that they may rarely, if ever, experience under natural conditions of exposure. In contrast, other lithologies (such as the basalt), which frequently reach high temperatures in the natural environment, may not attain these in laboratory tests. As a consequence, test blocks used in wetting and drying tests may dry at a slower rate and may not dry out completely over the duration of a test cycle. Where salts are present, a slower drying rate may result in crystallization at the surface as an efflorescence, compared to rapid heating or drying under natural conditions, which is more likely to induce crystallization at depth. Crystallization at depth is more likely to produce phenomena such as flaking and scaling (Smith and McGreevy 1988), whereas surface crystallization is more commonly associated with granular disaggregation. Obviously, incomplete drying of the samples will also limit the overall crystallization of any salts within a stone.
The significance of these observations lies in their implications for (1) the relative effectiveness of mechanical weathering processes, and (2) the overall assessment of stone durability through exposure to these processes in oven-based tests.
Laboratory evidence reported in the open literature points to a positive relationship between temperature and the effectiveness of mechanical weathering such as salt crystallization (Price 1978; Sperling and Cooke 1985; Davison 1986; Goudie 1993). High stone temperatures may enhance breakdown by increasing salt crystallization pressures (Winkler and Singer 1972; Sperling and Cooke 1980) and concentrating salts in surface and near-surface layers. Consequently, in oven-based tests, indirect heating may contribute to more serious deterioration of, for example, samples with a relatively high albedo because they will be forcibly cycled through uncharacteristically high surface and subsurface temperature regimes. Conversely, salt crystallization may appear to be less effective in lithologies with a low albedo because the high surface-subsurface temperature frequently experienced in the natural environment may not be replicated under laboratory conditions. However, given that the majority of laboratory tests employ quite high temperatures (Marschner 1978; Ross and Butlin 1989, crystallization test), it is probable that most have tended to “overweather” high albedo stone types.
Disparities are often reported between the durability of stone as indicated by laboratory tests and behavior once exposed to “real world” conditions. Given that stone deterioration reflects a complex interaction between stone properties and environment, such disparities may well derive from the use of unrealistic parameters and/or the exclusion of relevant components in experimental design: failure to include the effect of thermal properties through the use of indirect heating methods is an example. Any comparative assessment of susceptibility to, for example, salt weathering, made on the basis of such conditions will not provide a true measure of weathering effectiveness or stone susceptibility. If direct heating of specimens is not possible, each stone type should be assessed separately using indirect heating regimes that reflect temperatures experienced under natural conditions, i.e., they should be both site- and stone-specific. Only through the inclusion of all properties that control the interaction of stone with other deterioration factors can more accurate predictions of behavior under a given set of conditions be made.
Finally, and specifically, the results of the soiling experiments add to the conservation debate as to whether features such as the pollution-related black crusts and stains that typically develop on building surfaces infrequently exposed to rainwash should be removed. Previous arguments have centered around questions of aesthetics, historical integrity, cost, the need for consolidation of cleaned stone, and the role of crusts as repositories of salts that can migrate into the underlying stone (Maxwell 1992). Our data suggest that, for some stone types, removal of dark surface stains and soiling could at least reduce the degree of mechanical stressing that they experience as a result of temperature fluctuations, especially rapid short-term changes. Under such conditions, temperature fluctuations are most marked in the outer millimeter or so—the precise zone in which granular disaggregation occurs. This finding is of particular relevance to lithologies that comprise a variety of minerals with different thermo-elastic properties, but it also has implications for homogeneous varieties, such as marble, in which grain orientation is variable (Yatsu 1988; Lewin 1990).
Much scope remains for the collection of microenvironmental data from “soiled” and “clean” stone surfaces under “real world” conditions. Furthermore, in natural environments (unpolluted), especially in hot climates, the effect of albedo change may also be an important factor in stone deterioration. Development of rock varnishes (Dorn and Oberlander 1982; Whalley et al. 1984), weathering rinds (Anderson and Anderson 1981; Dorn et al. 1991), iron staining (Dorn 1998) and algal and lichen growth (Satterwhite et al. 1985; Urz� et al. 1993) can all alter stone surface coloration. Although the causes may differ from those prevalent in urban areas, the overall effect should be the same, i.e., more extreme temperature regimes at the stone surface.
5 5. CONCLUSIONS
“Research into stone conservation demands an interdisciplinary approach. Many researchers, however, find themselves working alone or in relatively small teams. As a result research can become too narrow, failing to take into account factors that might seem self-evident to somebody trained in another discipline” (Price 1996, 34).
We have viewed this article as a contribution to a research area that affords much potential for the interdisciplinary approach advocated by Clifford A. Price (1996). It is hoped that the results reported and the implications arising from them will be of interest and some relevance to conservation and conservation science perspectives on stone deterioration. Over the past century, geomorphologists have produced an abundant literature on many aspects of stone weathering, some within conservation contexts (Merrill 1900; Barton 1916; Emery 1960; Goudie 1977). In recent years, however, they have shown a welcome tendency to tap into the literature of other disciplines (e.g., Evans 1970; McGreevy and Whalley 1984; Yatsu 1988; Goudie and Viles 1997), a tendency that has undoubtedly brought many benefits. We would encourage others to do likewise.
REFERENCES
Anderson, L. W., and D. S.Anderson. 1981. Weathering rinds on quartz arenite clasts as a relative age indicator and the glacial chronology of Mount Timpanogos, Wasatch Range, Utah. Arctic and Alpine Research13:25–31.
Barton, D. C.1916. Notes on the disintegration of granite in Egypt. Journal of Geology24:382–93.
Davison, A. P.1986. An investigation into the relationship between salt weathering debris production and temperature. Earth Surface Processes and Landforms11:335–41.
Dorn, R. I.1998. Rock coatings: Developments in earth surface processes, no. 6. Amsterdam: Elsevier.
Dorn, R. I., and T. M.Oberlander. 1982. Rock varnish. Progress in Physical Geography6:317–67.
Dorn, R. I., F. M.Phillips, M. G.Zreda, E. W.Wolfe, A.J.T.Jull, P. W.Kubik, and P.Sharma. 1991. Glacial chronology of Mauna Kea, Hawaii, as constrained by surface exposure dating. National Geographic Research and Exploration7:456–71.
Douglas, G. R., J. P.McGreevy, and W. B.Whalley. 1983. Rock weathering by frost weathering processes. In Proceedings of the Fourth International Conference on Permafrost, vol. 2. Fairbanks, Ala.: University of Alaska. 244–48.
Emery, K. O.1960. Weathering of the Great Pyramid. Journal of Sedimentary Petrology30(1):140–43.
Evans, I. S.1970. Salt crystallization and rock weathering: A review. Revue de G�omorphologie Dynamique19(4):153–77.
Geiger, R.1965. The climate near the ground. Cambridge, Mass.: Harvard University Press.
Goudie, A. S.1977. Sodium sulphate weathering and the disintegration of Mohenjo—Daro, Pakistan. Earth Surface Processes and Landforms2:75–86.
Goudie, A. S.1993. Salt weathering simulation using a single immersion technique. Earth Surface Processes and Landforms18:369–76.
Goudie, A. S., and H. A.Viles. 1997. Salt weathering hazards. Chichester, England: John Wiley & Sons.
Jenkins, K. A., and B. J.Smith. 1990. Daytime rock surface temperature variability and its implications for mechanical rock weathering: Tenerife, Canary Islands. Catena17:449–59.
Kerr, A., B. J.Smith, W. B.Whalley, and J. P.McGreevy. 1984. Rock temperatures from southeast Morocco and their significance for experimental rock weathering studies. Geology12:306–9.
Leary, E.1986. The building sandstones of the British Isles. Building Research Establishment Report, Department of the Environment, BRE, HMSO, London.
Lewin, S.1990. Susceptibility of calcareous stones to salt weathering. In First International Symposium on Conservation of Monuments in the Mediterranean Basin, ed. F.Zezza. Bari, Italy: Grafo Brescia. 59–63.
Maekawa, S., and N. H.Agnew. 1996. Investigation of environmentally driven deterioration of the Great Sphinx and concepts for protection. In Archaeological conservation and its consequences, ed. A.Roy and P.Smith. London: International Institute for Conservation of Historic and Artistic Works. 116–20.
Maekawa, S., F.Lambert, and J.Meyer. 1995. Environmental monitoring at Tiwanaku. In Materials issues in art and archaeology, vol. 4, ed. P. B.Vandiver et al.Pittsburgh: Materials Research Society. 885–92.
Marschner, H.1978. Application of salt crystallization test to impregnated stones. In RILEM/UNESCO International Symposium on Deterioration and Protection of Stone Monuments, Paris, report 3.4. Paris: RILEM, UNESCO.
Maxwell, I.1992. Stone cleaning—for better or worse? An overview. In Stone cleaning and the nature, soiling and decay mechanisms of stone, ed. R.G.M.Webster. London: Donhead Publishing. 3–49.
McGreevy, J. P., and W. B.Whalley. 1984. Weathering. Progress in Physical Geography8:541–69.
Merrill, G. P.1900. Sandstone disintegration through the formation of interstitial gypsum. Science11(283):850–51.
Price, C. A.1978. The use of the sodium sulphate crystallization test for determining the weathering resistance of untreated stone. In Deterioration and protection of stone monuments, report 3.6. Paris: RILEM, UNESCO.
Price, C. A.1996. Stone conservation: An overview of current research. Santa Monica, Calif.: Getty Conservation Institute.
Ross, K. D., and R. N.Butlin. 1989. Durability tests for building stone. Watford, England: Building Research Establishment (BRE).
Satterwhite, M. B., J.Ponder Henley, and J. M.Carney. 1985. Effects of lichens on the reflectance spectra of granitic rock surfaces. Remote Sensing of Environment18:105–12.
Smith, B. J.1977. Rock temperature measurements from the Northwest Sahara and their implications for rock weathering. Catena4:41–63.
Smith, B. J.1994. Weathering processes and forms. In Geomorphology of desert environments, ed.A. D.Abrahams and A. J.Parsons. London: Chapman and Hall. 39–63.
Smith, B. J., and R. W.Magee. 1990. Granite weathering in an urban environment: An example from Rio de Janeiro. Singapore Journal of Tropical Geography2:143–53.
Smith, B. J., and J. P.McGreevy. 1988. Contour scaling of a sandstone under simulated hot desert conditions. Earth Surface Process and Landforms13:697–706.
Sperling, C.H.B., and R. U.Cooke. 1980. Salt weathering in arid environments. Part 1, Theoretical considerations. Bedford College Papers in Geography8. London: University of London.
Sperling, C.H.B., and R. U.Cooke1985. Laboratory simulation of rock weathering by salt crystallization and hydration processes in hot arid environments. Earth Surface Processes and Landforms10:541–55.
Thorn, A.1994. Direct measurements to determine sandstone deterioration. In Preventive conservation practice, theory and research, ed. A.Roy and P.Smith. London: International Institute for Conservation of Historic and Artistic Works. 85–89.
Thorn, A., and A.Piper. 1996. The Isle of the Dead: An integrated approach to the management and natural protection of an archaeological site. In Archaeological conservation and its consequences, ed. A.Roy and P.Smith, London: International Institute for Conservation of Historic and Artistic Works. 188–92.
Urz�, C., G.Criseo, W. E.Krumbein, U.Wollenzien, and A. A.Gorbushina. 1993. Are colour changes of rocks caused by climate, pollution, biological growth or by interactions of the three? In Conservation of stone and other materials, ed. M. J.Thiel. London: E & FN Spon.
Warke, P. A.1994. Inheritance effects in the weathering of debris under hot arid conditions. Ph.D. diss., Queen's University of Belfast.
Warke, P. A., and B. J.Smith. 1998. Effects of direct and indirect heating on the validity of rock weathering simulation studies and durability tests. Geomorphology22:347–57.
Warke, P. A., B. J.Smith, and R. W.Magee. 1996. Thermal response characteristics of stone: Implications for weathering of soiled surfaces in urban environments. Earth Surface Processes and Landforms21:295–306.
Whalley, W. B., J. P.McGreevy, and R. I.Ferguson. 1984. Rock temperature observations and chemical weathering in the Hunza region, Karakoram: Preliminary data. In Proceedings of the International Karakoram Project vol. 2. Cambridge: Cambridge University Press. 365–73.
Winkler, E. M., and P. C.Singer. 1972. Crystallization pressure of salts in stone and concrete. Geological Society of America Bulletin83:3509–14.
Yatsu, E.1988. The nature of weathering. Tokyo: Sozosha.
AUTHOR INFORMATION
JIM McGREEVY has been head of conservation at the Ulster Museum, Museums and Galleries of Northern Ireland, since 1991. He received a Ph.D. in geography from the Queen's University Belfast in 1982 and held postdoctoral positions at University College London and the Institute of Irish Studies, Belfast. From 1984 to 1991, he was a research assistant at the Public Record Office of Northern Ireland, where he had responsibility for the paper conservation section. He is currently an Honorary Research Fellow in the School of Geography at Queen's University. His research has focused mainly on relationships between stone properties and susceptibility to weathering under various environmental conditions. Address: Department of Conservation, Ulster Museum, Museums and Galleries of Northern Ireland, Botanic Gardens, Belfast BT9 5AB, Northern Ireland, United Kingdom
PATRICIA A. WARKE is a lecturer in geomorphology at the School of Geography, Queen's University, Belfast. Her Ph.D., which was awarded in 1994, involved field study of rock weathering in the deserts of the Southwest United States. From 1994 to 1999, until her appointment as lecturer, she held a postdoctoral position in the School of Geography, which mainly entailed investigation of stone decay on historic structures in urban environments. Research interests are primarily focused on microenvironmental controls on rock weathering in both natural and built environments and the role of exposure history on contemporary stone decay exemplified by recent work on deterioration of newly excavated archaeological stonework. Address: School of Geography, Queen's University Belfast, Belfast BT7 1NN, Northern Ireland, United Kingdom
BERNARD J. SMITH is professor of tropical geomorphology in the School of Geography, Queen's University, Belfast, where he has worked since 1979. Previously, he lectured in northern Nigeria after obtaining both his undergraduate degree and Ph.D. at the University of Reading, England. His main research interest is the study of stone decay processes in natural and built environments. He has published widely on desert weathering, on deep weathering in the humid tropics, and on the weathering of limestones around the Mediterranean. Work on urban stone decay, particularly related to salt damage, has encompassed studies in Venice, Budapest, Rio de Janeiro, Dublin, and, in particular, Belfast. Address as for Warke
Received for review December 21, 1998. Revised manuscript received June 21, 1999. Accepted for publication January 20, 2000.
SOURCES OF MATERIALSHeat Sink CompoundRS Components, Ltd. ElectrolubeWentworth House
Blakes Raod, Wargrave
Berkshire RG10 8AW
United Kingdom
Section Index |